Diffusion Rate: Key Factors And Optimization For Enhanced Delivery
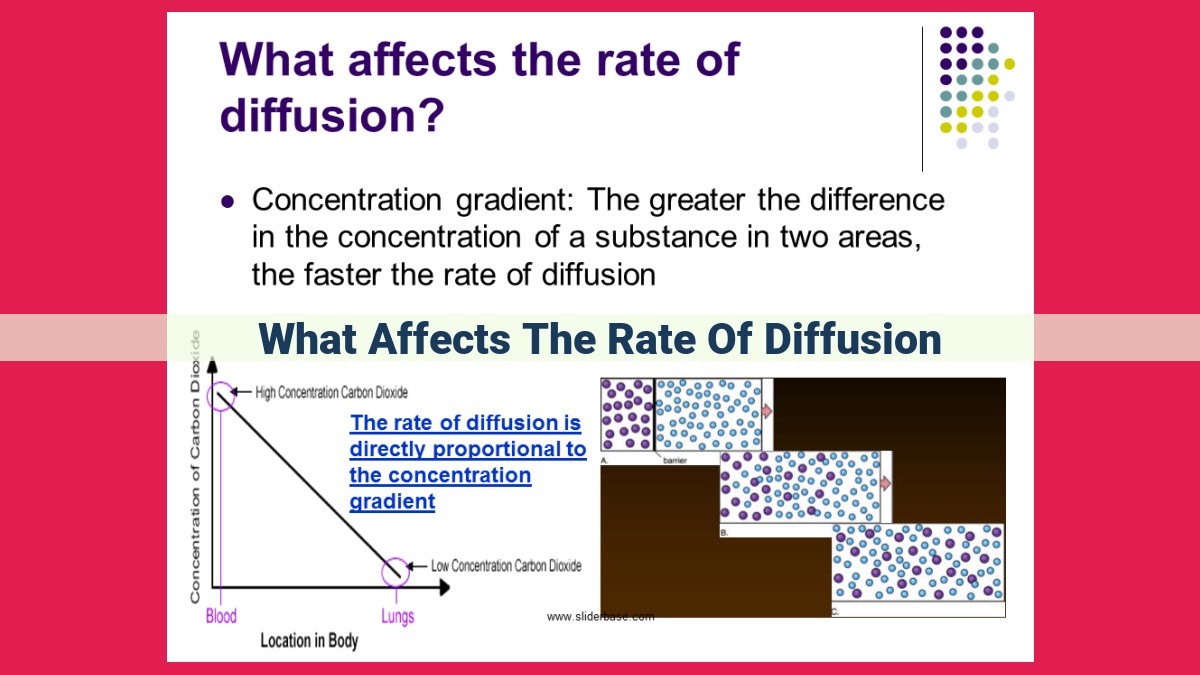
The rate of diffusion is influenced by several factors including concentration gradient (driving force), temperature (particle kinetic energy), surface area (diffusion channels), membrane thickness (resistance barrier), particle size (mass), lipid solubility (membrane permeability), and electric charge (electrostatic interactions). A higher concentration gradient, increased temperature, larger surface area, thinner membranes, smaller particles, greater lipid solubility, and favorable charge distribution promote faster diffusion.
Concentration Gradient: The Driving Force of Diffusion
In the world of molecules, movement is everything. Diffusion is the dance they perform when they want to spread their presence far and wide. But what drives these tiny dancers? Enter the concentration gradient.
Imagine a backyard with kids scattered about. Now, imagine some delicious cookies in the kitchen. What do you think will happen? The kids will naturally move from an area with fewer cookies (low concentration) to an area with more cookies (high concentration). That’s the driving force of diffusion!
Molecules act like these kids. When there’s a higher concentration gradient, they sense the difference and move from a place where they’re less crowded to a place where there’s more room to move. The bigger the concentration difference, the faster they diffuse.
For instance, think of a drop of perfume in a room. The perfume molecules diffuse from the drop’s high concentration to the lower concentration in the room, spreading the scent throughout the space. It’s the concentration gradient that makes it happen!
Diffusion: The Dance of Molecules in Motion
Diffusion is the backbone of life, the driving force behind the everyday processes that keep us alive. An unseen dance of molecules, this phenomenon plays a crucial role in everything from the exchange of oxygen in our lungs to the absorption of nutrients in our bloodstream.
Temperature: The Conductor of Molecular Energy
Temperature, like a maestro, conducts the symphony of molecular motion. As the temperature rises, kinetic energy—the energy of particle movement—soars. This increased energy becomes the fuel that drives molecules to move faster and more frequently. The higher the kinetic energy, the more collisions occur, and the quicker particles diffuse. Imagine a crowded dance floor; the more energetic the dancers, the more they bump into each other, creating a lively and fast-paced dance.
In the realm of diffusion, faster particle movement translates to a more rapid spread of molecules. Substances can dance across membranes and travel through tissues with ease. This heightened diffusion rate ensures that essential molecules reach their destinations swiftly, keeping the body’s machinery humming.
Surface Area: Maximizing the Diffusion Pathway
- Explain the importance of surface area for providing more diffusion channels.
- Provide examples of how increasing surface area enhances diffusion rates.
Surface Area: The Diffusion Booster
Picture this: you’re trying to fill a bathtub with water. How do you get it done faster? By increasing the surface area of the faucet! The same principle applies to diffusion, the process by which molecules move from an area of high concentration to an area of low concentration. Surface area plays a crucial role in maximizing the diffusion pathway, allowing for a more efficient exchange of molecules.
Imagine a factory with only one gate. Now, imagine the same factory with multiple gates. Obviously, the factory with more gates will allow more goods to enter and leave, right? Similarly, in diffusion, a larger surface area provides more diffusion channels, allowing more molecules to pass through at once.
For example, the lungs have a vast surface area, with alveoli (tiny air sacs) that provide a huge number of diffusion channels for oxygen and carbon dioxide molecules to exchange between the lungs and the blood. Likewise, the small intestine has numerous villi and microvilli, which increase its surface area and allow for faster absorption of nutrients from the food we eat.
Increasing surface area is not just limited to biological systems. Engineers have designed various devices and materials to enhance diffusion rates. For instance, catalytic converters in cars have a honeycomb structure with a large surface area, which maximizes the contact between exhaust gases and the catalyst, leading to more efficient removal of pollutants.
Remember, when it comes to diffusion, surface area is king. The greater the surface area available, the quicker molecules can travel from one place to another. So, the next time you’re trying to speed up a diffusion process, think about ways to increase the surface area and watch the molecules flow!
Membrane Thickness: The Resistance Barrier to Diffusion
Diffusion, the process by which particles move from an area of high concentration to low concentration, is essential for various biological processes. However, the rate of diffusion can be affected by several factors, including membrane thickness.
Membranes are barriers that separate different compartments within cells. They play a crucial role in controlling the movement of substances into and out of cells. The thickness of a membrane can significantly influence how easily particles can diffuse across it.
** thicker membranes hinder particle movement**. This is because particles have to travel a longer distance to cross the membrane, increasing the resistance to their movement. As a result, the rate of diffusion decreases with increasing membrane thickness.
The properties of the membrane can also affect the rate of diffusion. For instance, membranes that are less fluid or have a higher lipid content can be more resistant to diffusion. This is because less fluid membranes impede particle movement, while lipids can interact with particles and slow their passage.
Understanding the role of membrane thickness in diffusion is important for comprehending cellular processes. For example, in the lungs, the thin alveolar walls allow for efficient diffusion of oxygen from the air into the bloodstream. In contrast, the thick blood-brain barrier restricts the entry of certain substances into the brain, protecting it from potentially harmful molecules.
In summary, membrane thickness acts as a resistance barrier to diffusion. Thicker membranes and certain membrane properties can slow down the movement of particles across them. Recognizing the influence of membrane thickness is crucial for elucidating the dynamics of diffusion in biological systems.
Particle Size: Size Matters in Diffusion
In the realm of chemistry and biology, diffusion plays a pivotal role in facilitating the movement of substances across concentration gradients. One fascinating aspect of diffusion is the profound influence that particle size exerts on its rate. Smaller particles glide through the molecular landscape with remarkable ease, leaving their larger counterparts trailing behind.
The secret to this disparity lies in the fundamental principles of particle motion. As particles shrink in size, their mass diminishes. This reduction in mass translates into higher kinetic energy, which is the energy of motion. With greater kinetic energy, smaller particles possess a heightened ability to overcome obstacles and navigate through the molecular maze.
Moreover, the surface area to volume ratio of particles drastically changes as their size decreases. Smaller particles boast a larger surface area relative to their volume, which means they interact more effectively with the surrounding environment. This enhanced surface area allows for more efficient collisions and facilitates a swift movement of particles.
For instance, consider the diffusion of oxygen molecules in the human body. Oxygen, being a small molecule, can effortlessly penetrate cell membranes to reach the mitochondria, where it is essential for cellular respiration. In contrast, larger molecules, such as glucose, require specialized channels or transporters to cross the membrane due to their hefty size and reduced surface area.
Understanding the impact of particle size on diffusion is critical in various fields. In drug delivery, for example, smaller drug molecules can penetrate tissues and target specific cells more effectively. In nanotechnology, the controlled synthesis of nanoparticles with tailored sizes enables the development of novel materials with unique properties and applications.
In summary, particle size matters profoundly in diffusion. Smaller particles, armed with higher kinetic energy and a larger surface area to volume ratio, dance through the molecular terrain with remarkable agility. This understanding has far-reaching implications in fields as diverse as biology, medicine, and materials science.
Lipid Solubility: A Passageway through Membranes
When molecules encounter a cell membrane, their journey across can be akin to navigating a guarded fortress. However, certain molecules possess a key that unlocks this barrier: lipid solubility.
Lipids, the building blocks of membranes, are molecules that dissolve in organic solvents. This property allows them to form a hydrophobic (water-repelling) barrier that surrounds cells. For molecules to penetrate this barrier, they too must be lipid-soluble, meaning they can dissolve in lipids.
Molecules with a high lipid solubility can easily slip through the membrane’s lipid bilayer. This is because the nonpolar tails of these molecules align with the hydrophobic interior of the membrane, while their polar heads interact with the membrane’s polar surface. This alignment creates a “greasy chute” that allows molecules to cross the membrane with ease.
Conversely, molecules that are hydrophilic (water-loving) are repelled by lipids. These molecules cannot dissolve in the membrane and are unable to penetrate it. This limited access can have significant implications for cellular function.
For example, oxygen is a vital gas that cells require for respiration. Oxygen is lipid-soluble and can easily diffuse across cell membranes to reach its intracellular destination. In contrast, sodium ions are hydrophilic and cannot diffuse across the hydrophobic membrane. Instead, they rely on specialized channels or pumps to transport them across.
The lipid solubility of molecules is therefore a crucial factor in determining their ability to enter or leave cells. It plays a central role in the exchange of nutrients, waste products, and signaling molecules, ultimately influencing the overall function of cells and tissues. Remember, when it comes to crossing cell membranes, lipid solubility is the golden ticket.
Diffusion’s Dance: The Influence of Charge
In the realm of biology, diffusion reigns as a fundamental process, governing the movement of particles across membranes. Just as dancers respond to the rhythm and flow of music, particles heed the call of concentration gradients, kinetic energy, and molecular properties in their diffusion journey.
Among these factors, charge, like an electrostatic choreographer, plays a captivating role in dictating particle movement. Electric charge, the inherent property of particles to carry a positive or negative electrical potential, profoundly influences their passage through membranes.
Cell membranes, the gatekeepers of our cells, are adorned with a lipid bilayer, a hydrophobic barrier that selectively allows molecules to pass. However, for charged particles, this membrane presents a formidable obstacle.
Positively charged particles face a positive barrier, as like charges repel. This repulsion hinders their diffusion across the membrane, slowing their journey. Conversely, negatively charged particles encounter an attractive force, drawn towards the positive charges within the membrane. This attraction accelerates their diffusion, allowing them to flow more freely.
Membrane potential, the electrical gradient across the membrane, further modulates the dance of charged particles. A positive membrane potential further inhibits cation diffusion (the movement of positively charged particles), while a negative membrane potential enhances it. Similarly, the diffusion of anions (negatively charged particles) is promoted by a positive membrane potential and hindered by a negative one.
In summary, the interplay between charge and membrane properties paints an intricate choreography of diffusion. Positively charged particles waltz meticulously across the membrane, while negatively charged particles pirouette with ease. The rhythm of their movement harmonizes with the electrical symphony of the cell, ensuring the seamless flow of molecules across the membrane barrier.