Understand Diffusion: The Key To Particle Distribution And Biological Processes
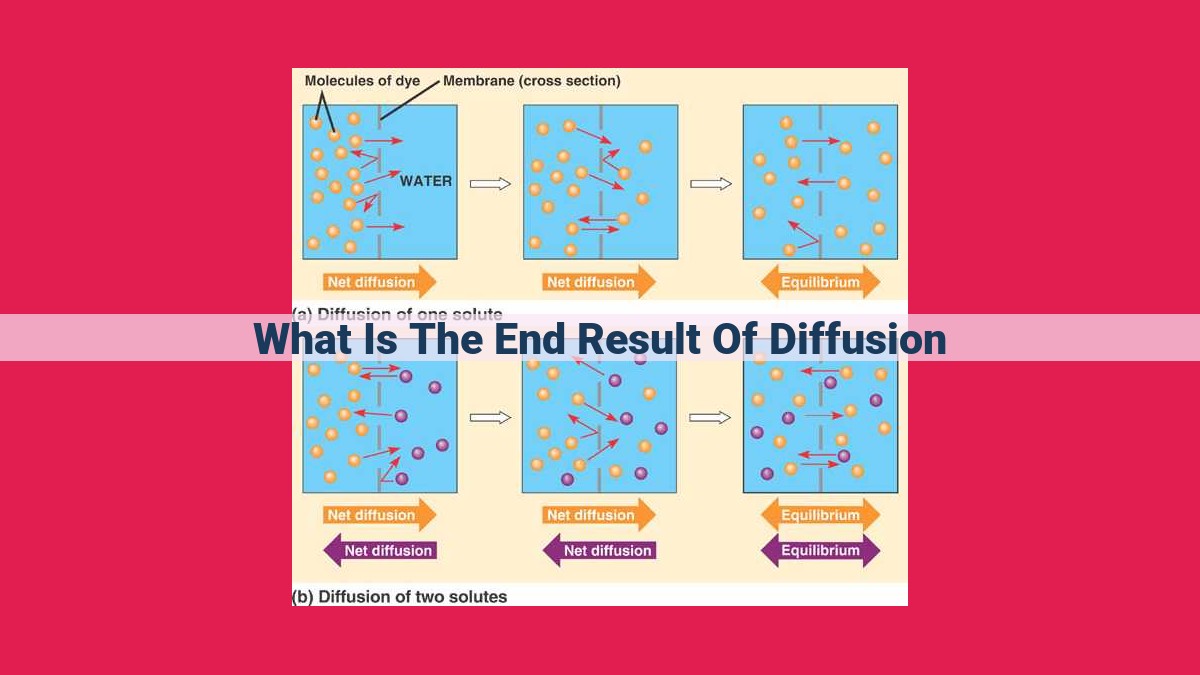
Diffusion, the movement of molecules across concentration gradients, results in the equitable distribution of particles throughout a space, eliminating concentration gradients. As particles randomly move due to Brownian motion, there is a net movement from high to low concentration areas, leading to equilibrium. Equilibrium is reached when particle motion is balanced by concentration gradients, ensuring the distribution of particles across biological membranes and facilitating essential biological processes.
Diffusion: The Unseen Dance of Molecules
In the microscopic realm of life, there exists a fascinating phenomenon called diffusion, an invisible but crucial process that drives countless biological functions. When you breathe in oxygen or taste a sip of coffee, diffusion is the silent choreographer behind these life-sustaining events.
What is Diffusion?
Imagine a crowd of people milling about in a room. If one corner of the room suddenly becomes crowded, the people in that area will start to disperse to the less crowded areas. This movement of people is like diffusion in the molecular world. Molecules, like tiny particles, move from areas of higher concentration (i.e., more molecules) to areas of lower concentration.
Why is Diffusion Important?
Diffusion is essential for countless biological processes. It allows oxygen to reach our cells, nutrients to spread throughout our bodies, and waste products to be removed. Without diffusion, our organs would suffocate, and our bodies would quickly become toxic.
In the microscopic world, diffusion operates tirelessly, ensuring that molecules reach their intended destinations. It’s a fundamental force that supports the delicate balance of life, ensuring that all of our cells have the molecules they need to function properly.
Understanding Concentration Gradient
- Concept of concentration gradient as the difference in concentration between two areas.
- How concentration gradient drives diffusion.
Understanding Concentration Gradient
Imagine a room filled with a sweet fragrance. As you step into the room, you can immediately sense the scent. This is because the molecules of the fragrance are in motion, spreading out evenly throughout the space. The process that drives this movement is called diffusion, and it all begins with a concentration gradient.
A concentration gradient is the difference in the concentration of a substance between two areas. In the case of our fragrant room, the concentration of fragrance molecules is higher near the source of the scent and lower further away. This difference in concentration creates a driving force that propels the fragrance molecules from areas of high concentration to areas of low concentration.
This movement is not a direct path, but rather a random walk. The fragrance molecules bounce around, colliding with other molecules and objects in their path. However, over time, the net movement of these molecules is from high to low concentration. This net movement continues until the concentration is the same everywhere in the room, at which point equilibrium is reached.
Net Movement of Particles: The Driving Force of Diffusion
In the realm of molecules, a constant dance of movement occurs, driven by the invisible yet powerful force of diffusion. This natural process ensures that molecules spread out, moving from areas of high concentration to areas of low concentration. It’s a continuous ballet of molecular motion, with particles colliding and bouncing off each other in a chaotic but purposeful manner.
Random Motion: The Fuel of Diffusion
Imagine a crowd of people jostling and weaving through a crowded street. Each individual moves randomly, colliding with others and changing direction countless times. This seemingly chaotic motion is essential for diffusion because it allows molecules to explore their surroundings. As they bounce around, they encounter areas with varying concentrations of other molecules.
From High to Low: The Concentration Gradient’s Influence
Now, let’s introduce a concentration gradient—a difference in the concentration of molecules between two areas. This gradient creates an imbalance, driving the net movement of particles. Think of it like water flowing downstream: molecules move from the area with a higher concentration, where they are more crowded, to the area with a lower concentration, where they have more space to spread out.
A Constant Flux: Overcoming Randomness
Despite the constant random motion, the net movement of particles is always from high to low concentration areas. This is because the concentration gradient exerts a directional force on the molecules, guiding their overall movement. Over time, this net movement leads to a more even distribution of molecules, eventually reaching a state of equilibrium.
Equilibrium: The Balancing Act of Diffusion
As molecules embark on their diffusive journey, a crucial point awaits them—equilibrium. In this state of serene balance, the driving force behind diffusion, the concentration gradient, fades into oblivion. Molecules no longer exhibit a net movement towards any particular direction, creating a harmonious equilibrium where concentration levels remain constant throughout.
At equilibrium, the random motion of particles—often observed as Brownian motion—ceases to favor one direction over another. The ceaseless dance of molecules remains, but it becomes symmetrical. Imagine a crowd of people milling about a room, their movements a chaotic symphony. At equilibrium, the number of people entering and leaving any given area remains equal, resulting in no discernible flow or direction.
This state of equilibrium is essential in countless biological processes. For instance, it allows cells to maintain their proper concentration gradients of essential substances, such as ions and nutrients. Without equilibrium, these gradients would dissipate, disrupting cellular processes and potentially leading to cellular dysfunction.
Thus, equilibrium serves as the ultimate destination for diffusion, a point where the driving forces of concentration gradients and random molecular motion harmonize to create a state of stability. It is a testament to the delicate balance that governs the microscopic world within our cells and beyond.
Brownian Motion: The Zigzag Journey of Particles Facilitating Diffusion
In the fascinating world of diffusion, particles embark on a random dance, propelled by the invisible forces of concentration gradients. This dance, known as Brownian motion, plays a crucial role in facilitating diffusion, the essential process that drives the movement of molecules across biological systems.
Imagine a swarm of particles suspended in a fluid, like tiny pollen grains in a gentle breeze. Each particle undergoes constant, random motion, colliding with its neighbors and bouncing off in unpredictable directions. This chaotic movement creates a zigzag path, reminiscent of the Brownian motion observed by the botanist Robert Brown in 1827.
The Brownian motion of particles is what drives diffusion. When there is a concentration gradient, a difference in concentration between two areas, the particles randomly move from areas of high concentration to areas of low concentration. As they do so, they carry with them their chemical energy, effectively equalizing the concentration across the gradient.
This process continues until equilibrium is reached, a state where the concentration gradient is eliminated. At this point, the net movement of particles ceases, and the system reaches a balance between random motion and the concentration gradient.
Brownian motion is essential for a multitude of biological processes, such as the absorption of nutrients and the transport of waste products. Without this random dance of particles, the cells and organs of our bodies would struggle to function properly.
So, next time you see a suspended particle moving in a seemingly erratic manner, remember that it is not simply a chaotic movement. It is Brownian motion, a fundamental force that drives the essential process of diffusion and supports life as we know it.