Diaphragm In Microscopy: Enhancing Image Quality And Precision
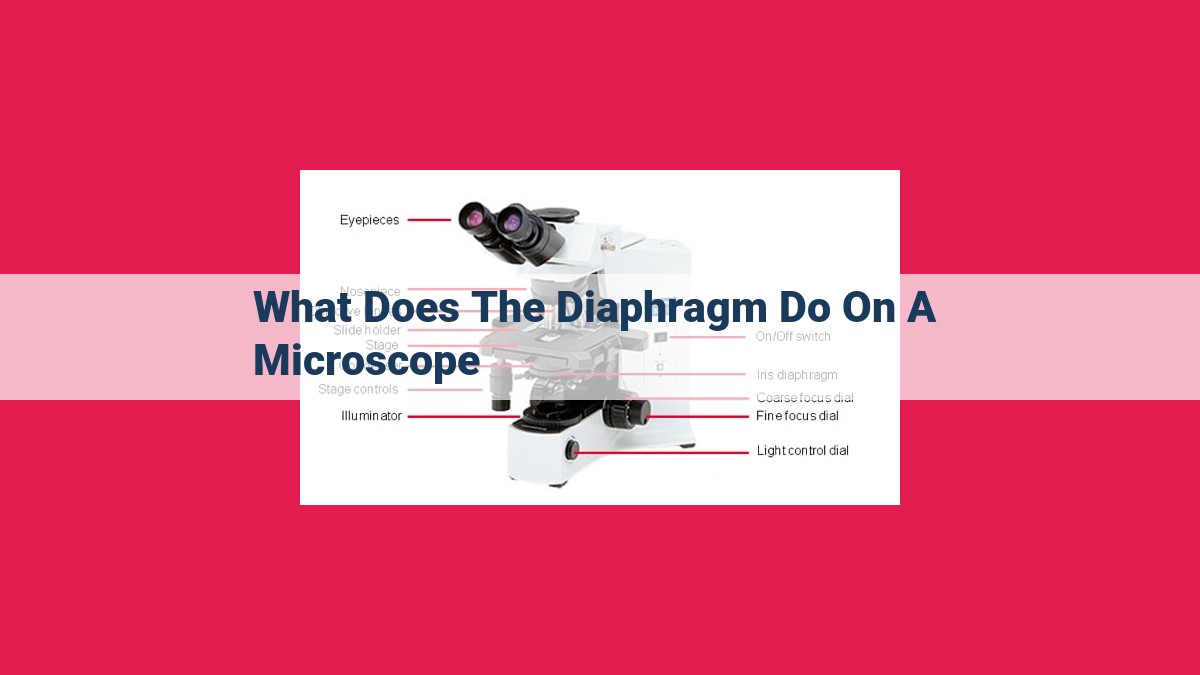
The diaphragm in microscopy controls the intensity and distribution of light passing through the specimen, enhancing image quality and clarity. By adjusting the aperture size, the diaphragm regulates light intensity, exposure time, and contrast. It also manipulates depth of field, highlighting specific structures and optimizing resolution. Furthermore, the diaphragm influences the effectiveness of additional techniques such as polarizing filters, darkfield illumination, and differential interference contrast, which enhance visibility and enable targeted observation of cellular components.
The Diaphragm: A Master of Light in Microscopy
In the realm of microscopy, the diaphragm stands as a crucial player, orchestrating the delicate balance of light to reveal the hidden wonders of the microscopic world. Its primary role lies in modulating the intensity of the light illuminating the specimen, akin to a conductor adjusting the volume of a symphony orchestra. By regulating the aperture size, exposure time, and light source intensity, the diaphragm empowers microscopists with unmatched precision in crafting images of unparalleled clarity and detail.
Manipulating Aperture Size for Optimal Illumination
The aperture, a circular opening within the diaphragm, dictates the diameter of the light beam passing through the condenser and objective lens. By adjusting the aperture size, microscopists can fine-tune the intensity of illumination. A larger aperture admits more light, resulting in a brighter image but potentially sacrificing resolution. Conversely, a smaller aperture reduces illumination, leading to a dimmer image but enhancing resolution. Striking the perfect balance between brightness and resolution is paramount for capturing images that unveil the intricacies of the specimen.
Calibrating Exposure Time for Accurate Imaging
The exposure time refers to the duration that the light source illuminates the sample. This parameter is critical in determining the overall brightness of the image. By increasing the exposure time, more light is gathered, resulting in a brighter image. However, excessively long exposure times can lead to image blurring, especially when observing dynamic processes within living cells. On the other hand, shorter exposure times reduce image brightness but minimize blurring, making them ideal for capturing fast-moving cellular events.
Adjusting Light Source Intensity for Specialized Techniques
The light source intensity can be adjusted to accommodate specific microscopy techniques. For instance, high-intensity illumination is essential for fluorescence microscopy, a powerful technique that utilizes fluorescent dyes to visualize specific molecules within cells. Conversely, low-intensity illumination is preferred for phase-contrast microscopy, which enhances the visibility of transparent specimens by manipulating the phase of light waves. By tailoring the light source intensity to the needs of the microscopy technique, researchers can optimize their observations and unravel the hidden secrets of the cellular realm.
Diaphragm in Microscopy: A Comprehensive Guide
In the realm of microscopy, where the unseen world reveals its secrets, the diaphragm reigns supreme as a master of illumination. This unassuming yet crucial component holds the key to unlocking the intricacies of microscopic specimens, empowering scientists and enthusiasts alike to unravel the wonders hidden within.
To comprehend the diaphragm’s pivotal role, we must first delve into the nature of light and its pivotal role in microscopy. Light, a radiant symphony of electromagnetic waves, carries vital information about the specimen being observed. When harnessed effectively, light can illuminate the tiniest structures, revealing their shapes, textures, and interactions.
The diaphragm, nestled within the microscope’s condenser, acts as a gatekeeper of light. By controlling the aperture size, the diaphragm regulates the intensity of light passing through the specimen. A wider aperture allows more light to enter, resulting in a brighter image, while a narrower aperture restricts the light, producing a dimmer image. This control over brightness is essential for ensuring optimal image exposure, preventing overexposure or underexposure that could obscure crucial details.
Moreover, the diaphragm influences the light source intensity, further fine-tuning the illumination. A brighter light source yields a brighter image, while a dimmer source produces a dimmer image. By adjusting the aperture size and light source intensity in tandem, microscopists can achieve the ideal lighting conditions for their specific specimens.
These factors, working in concert, ultimately impact the brightness and detail of the microscopic image. By manipulating the diaphragm, microscopists can optimize illumination to reveal the intricate beauty and hidden secrets of the microscopic world.
Diaphragm in Microscopy: A Comprehensive Guide
The diaphragm is a crucial component of any microscope, playing a vital role in controlling light intensity, contrast, resolution, and depth of field. In this comprehensive guide, we’ll delve into the intricacies of the diaphragm and its essential functions in microscopy.
Manipulating Contrast and Resolution: A Dynamic Trio
The diaphragm, condenser, objective lens, and eyepiece work in concert to manipulate contrast and resolution, two fundamental qualities of a microscopic image. The condenser directs a focused beam of light onto the specimen, illuminating it. The objective lens gathers the transmitted or reflected light and magnifies the image, while the eyepiece further magnifies and corrects the image for viewing.
By adjusting the diaphragm, you can control the numerical aperture (NA) of the objective lens, which determines the depth of field and resolving power. The NA is directly proportional to the diameter of the light beam; a larger NA corresponds to a higher resolution but a shallower depth of field.
Fine-tuning Contrast: Condenser and Objective Lens
The condenser and objective lens play pivotal roles in controlling contrast. The condenser adjusts the angle and intensity of the illuminating light, affecting the brightness and darkness of the image. By manipulating the condenser’s position and diaphragm opening, you can enhance or reduce contrast, making specific structures more visible.
The objective lens’s numerical aperture also influences contrast. Lenses with higher NAs provide greater resolving power but reduced contrast. By carefully selecting the appropriate objective lens for your specimen, you can achieve an optimal balance between contrast and resolution.
Diaphragm in Microscopy: A Comprehensive Guide
1. Controlling Light Intensity for Optimal Imaging
The diaphragm in a microscope serves as a crucial gatekeeper, regulating the amount of light that reaches the specimen. By adjusting its aperture size, you can fine-tune the brightness and detail of your microscopic image. A smaller aperture reduces light intensity, increasing the contrast of your image while sacrificing brightness. Conversely, a larger aperture lets in more light, resulting in a brighter image but potentially lower contrast. The diaphragm also allows you to control exposure time, ensuring that your images capture the optimal amount of light.
2. Manipulating Contrast and Resolution for Enhanced Visualization
The diaphragm interacts seamlessly with other optical components in your microscope to enhance image contrast and resolution. The condenser, positioned beneath the stage, condenses the light source into a cone-shaped beam, illuminating your specimen. The objective lens then gathers and focuses this light, magnifying your specimen for observation. The interplay between the condenser and objective lens determines the angle of light striking your specimen, influencing the visibility and clarity of its features.
3. Adjusting Depth of Field for Comprehensive Analysis
The diaphragm plays a pivotal role in controlling the depth of field, the range of focus within your image. A narrower diaphragm limits the diameter of the light beam, increasing the depth of field. This allows you to observe a larger portion of your specimen in focus, making it ideal for examining the overall structure and organization of cells. Conversely, a wider diaphragm decreases the depth of field, providing a highly focused view of specific structures. This is useful for detailed analysis of subcellular components or studying changes in cell morphology.
Polarizing Filters: Enhancing Image Clarity in Microscopy
When peering into the microscopic world, clarity is paramount. Image clarity allows researchers to observe minute details, draw accurate conclusions, and make groundbreaking discoveries. One indispensable tool for achieving this clarity is the polarizing filter.
Polarizing filters are akin to sunglasses for your microscope. They selectively filter out light waves that vibrate in certain directions, reducing glare and unwanted reflections. This results in a dramatic improvement in image contrast, making it easier to discern subtle differences in the specimen.
Imagine you’re observing a pollen grain under a microscope. Without a polarizing filter, you may be overwhelmed by the bright reflections bouncing off the shiny surface. But when you insert a polarizing filter, the reflections are eliminated, revealing intricate patterns and details that were previously obscured.
Polarizing filters not only enhance contrast but also improve image sharpness. By reducing glare, they sharpen the outlines of structures, making them more visible and easier to analyze. This is particularly beneficial in microscopy techniques such as differential interference contrast (DIC), where precise delineation of cellular components is crucial.
In summary, polarizing filters are an essential tool in the microscopist’s arsenal. They reduce glare, enhance contrast, and sharpen image details, allowing researchers to obtain clearer, more informative images of the microscopic world.
Brightfield and Darkfield Illumination: Sharpening Image Contrast
In the realm of microscopy, where the unseen world is brought to life, the interplay of light and optics plays a crucial role in unveiling the intricate details of specimens. Among the essential components of a microscope, the diaphragm stands as a master of contrast, subtly controlling the path of light to enhance the visibility and clarity of images.
Brightfield Illumination: Revealing Surface Structures
Imagine peering into a microscopic landscape illuminated by a bright, uniform light beam. This is the world of brightfield illumination, where the specimen’s absorbance and scattering properties create contrasting effects. The denser or more refractive areas, such as cell nuclei or pigmented organelles, appear dark, while less dense regions allow light to pass through more readily, resulting in a brighter appearance. Brightfield illumination is particularly useful for showcasing surface structures, cell morphology, and general tissue architecture.
Darkfield Illumination: Uncovering Hidden Depths
Shifting the spotlight to darkfield illumination, we enter a realm where the principle of reflection reigns supreme. Instead of directing light directly onto the specimen, this technique uses an inclined condenser to illuminate it from the side. Light that strikes the specimen’s surface is reflected towards the objective lens, while unreflected light is blocked by the diaphragm. This creates a dramatic effect, with shiny or reflective structures appearing bright against a dark background. Darkfield illumination excels in visualizing transparent specimens, revealing fine details such as the flagella of bacteria or the internal contours of cells.
Diaphragm in Microscopy: A Comprehensive Guide
The diaphragm is a crucial component of a microscope, responsible for controlling light intensity, contrast, resolution, and depth of field. By manipulating these settings, scientists can optimize the visibility and clarity of microscopic images, enabling them to study the intricate details of cells and other specimens.
Adjusting Depth of Field for Comprehensive Analysis
Depth of field refers to the range of distances within a specimen that appear in focus. The diaphragm plays a key role in controlling this parameter by altering the diameter of the light beam that illuminates the specimen.
A narrow light beam creates a large depth of field, allowing a thicker specimen to be in focus. This is ideal for observing the overall structure of a cell or tissue. Conversely, a wide light beam results in a shallow depth of field, focusing on a specific layer or detail within the specimen. This is useful for examining subsurface features or specific organelles.
Optimizing Depth of Field for Different Specimens
The appropriate depth of field depends on the specimen being studied. For large or thick specimens, such as plant tissues or animal embryos, a larger depth of field is necessary to capture the entire structure in focus. For smaller or flat specimens, such as bacteria or thin cell cultures, a shallower depth of field is sufficient to highlight specific features.
Influence of Objective Lens and Condenser
In addition to the diaphragm, the objective lens and condenser also influence the depth of field. Higher magnification objective lenses produce a shallower depth of field, while lower magnification objective lenses provide a larger depth of field. Similarly, a high condenser position results in a shallower depth of field, while a low condenser position yields a larger depth of field.
By understanding the role of the diaphragm in controlling the diameter of the light beam and the impact of this on depth of field, scientists can adjust their microscope settings to optimize the visualization and analysis of microscopic specimens. From large tissues to intricate organelles, the depth of field is a critical factor in capturing clear and informative images for scientific research and exploration.
Diaphragm in Microscopy: A Comprehensive Guide
Adjusting Depth of Field for Comprehensive Analysis
The Artist’s Eye:
Imagine yourself as a meticulous artist, carefully capturing the intricate details of a masterpiece. Your brushstrokes, like the light beam in a microscope, dance across the canvas, revealing each layer with precision. The depth of field, akin to your brushwork’s focus, ensures that every element within a certain vertical range is sharply defined, while distant objects fade into a soft blur.
The Diaphragm’s Symphony:
Just as you control the aperture of your brush to determine how much paint it deposits, the diaphragm in a microscope regulates the diameter of the light beam. A narrower aperture creates a deeper depth of field, akin to a finely tapered brush that paints with precise strokes. Conversely, a wider aperture reduces depth of field, similar to a boldly swept brush that covers a broader area.
Objective Lens and Condenser, the Guiding Lights:
The objective lens, the magnifying glass of the microscope, plays a crucial role in depth of field. Higher magnification lenses produce a shallower depth of field, while lower magnification lenses offer a wider field of view. The condenser, positioned beneath the stage, focuses the light beam onto the specimen. Its position influences the angle of illumination, which in turn affects depth of field. By adjusting the condenser’s height, you can optimize the balance between resolution and depth of field.
Capturing the Essence of Your Observations:
Understanding depth of field is paramount for capturing comprehensive microscopic images. It allows you to discern fine details in a specific layer of the specimen while keeping other regions in context. It’s like reading a three-dimensional book, where each page represents a different layer of observation. By manipulating the diaphragm, objective lens, and condenser, you can shift your focus from the surface to the depths of your microscopic world, revealing a symphony of hidden details.
Highlighting Specific Structures for Targeted Observation
In the realm of microscopy, the diaphragm takes on a pivotal role, not only in controlling light intensity and contrast but also in enhancing the visibility of specific cellular components. Specialized techniques, such as staining, fluorescence, and differential interference contrast (DIC), empower microscopists to unveil the intricacies of the cellular universe.
Staining introduces dyes or stains that bind selectively to specific molecular structures, such as DNA, proteins, or lipids. This enhances their visibility by creating a contrast between stained and unstained areas. The choice of stain depends on the target structure and the desired fluorescence or color.
Fluorescence microscopy takes staining a step further by utilizing fluorescent dyes that emit light when exposed to certain wavelengths. This amplifies the signal from the stained structures, making them even brighter and easier to visualize. By employing different wavelengths of light, researchers can selectively highlight multiple cellular components simultaneously.
Differential interference contrast (DIC) employs a prism system to enhance the contrast of transparent specimens by converting phase differences in the light passing through the sample into brightness differences in the image. This technique excels in revealing the three-dimensional architecture of cells and tissues, providing depth and detail to the microscopic view.
Utilizing these techniques in combination, microscopists can target specific cellular components, amplify their visibility, and unveil their spatial relationships with unprecedented precision. By harnessing the power of stains, fluorescence, and DIC, researchers can delve into the intricate world of cells and unravel the secrets of life at its most fundamental level.
Explain the principles and applications of these techniques in microscopy.
Diaphragm in Microscopy: A Comprehensive Guide
Imagine yourself as a photographer, meticulously adjusting your camera settings to capture the perfect picture. In the world of microscopy, the diaphragm plays a crucial role, just like the aperture on a camera. It regulates the intensity and direction of light to enhance the visibility and detail of microscopic images.
Controlling Light for Brilliance and Clarity
The diaphragm, located in the condenser, acts as a gatekeeper for light entering the microscope. By adjusting the aperture size, you can control the diameter of the light beam, which in turn affects the brightness and exposure of the image. A wider aperture allows more light to pass through, resulting in a brighter image, while a narrower aperture reduces light intensity for enhanced contrast and detail.
Manipulating Contrast and Resolution for Sharper Images
The diaphragm also works hand-in-hand with the condenser and objective lens to optimize contrast and resolution. By adjusting the condenser’s position, you can control the angle of light illumination, which affects the depth of field and sharpness of the image. The objective lens magnification also influences resolution, as higher magnification allows for closer examination of fine details.
Enhancing Clarity for Precision Observation
Various techniques can further enhance image clarity in microscopy. Polarizing filters minimize glare and improve contrast, while brightfield and darkfield illumination techniques create distinct visual effects. Brightfield illumination provides a bright background for dark specimens, while darkfield illumination highlights edges and contours, offering a three-dimensional effect.
Adjusting Depth of Field for Comprehensive Analysis
The diaphragm also plays a crucial role in controlling the depth of field, or the range of distances that are in focus. A narrower diaphragm produces a greater depth of field, allowing you to observe a wider portion of the specimen in focus. Conversely, a wider diaphragm creates a shallower depth of field, isolating specific layers or structures for targeted examination.
Highlighting Structures for Targeted Observation
To enhance the visibility of specific cellular components, various staining and illumination techniques are employed in microscopy. Stains differentially bind to specific molecules, creating color contrast that makes them stand out. Fluorescence microscopy utilizes fluorescent dyes that emit light when exposed to a specific wavelength, allowing for the targeted visualization of proteins and other biomolecules. Differential interference contrast (DIC) utilizes polarized light to create a three-dimensional effect, highlighting differences in the refractive index of cellular structures.