Cytochrome C: The Electron-Carrying Hemoprotein In Energy Metabolism
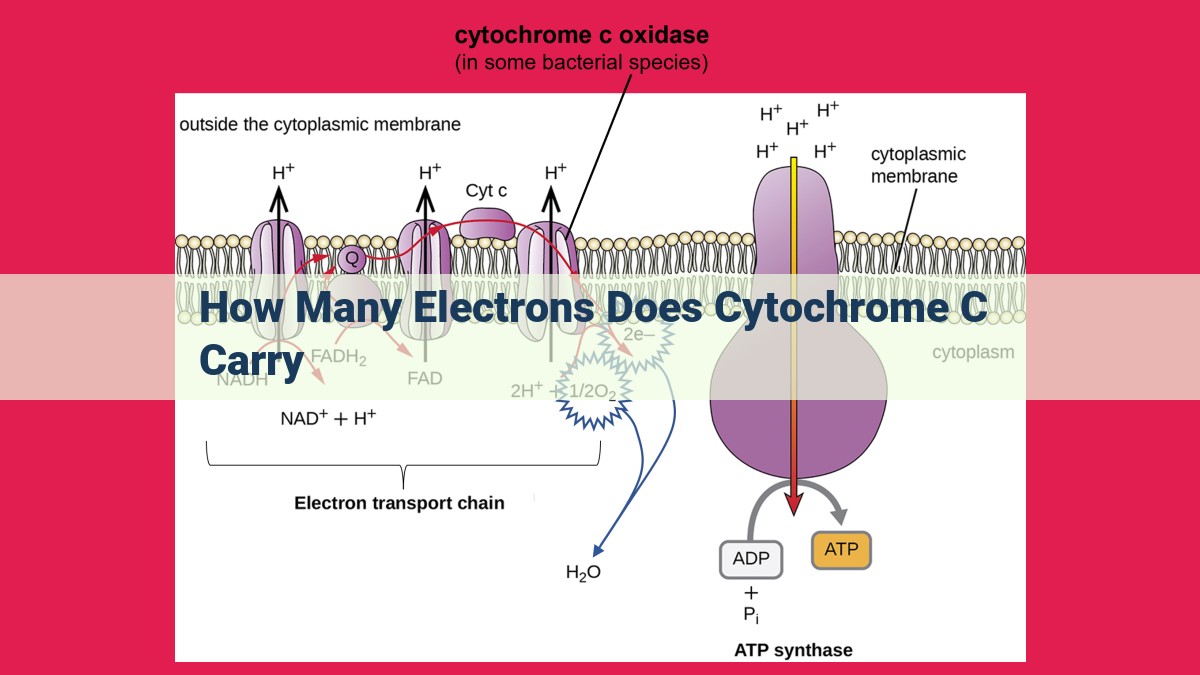
Cytochrome c, a hemoprotein involved in the electron transport chain, carries a single electron. This electron is bound to the iron ion within the heme group, which is a porphyrin ring complexed with iron. Cytochrome c’s electron-transfer function is crucial for oxidative phosphorylation, the process by which cells generate energy. Its role in the electron transport chain is similar to that of other hemoproteins, such as hemoglobin and myoglobin, which also carry electrons. Understanding electron transfer in cytochrome c is essential for comprehending cellular respiration and energy metabolism.
Understanding the Behavior of Electrons: Unraveling the Quantum Enigma
Electrons: The Fundamental Building Blocks of Matter
In the vast expanse of the universe, our world is built upon the intricate dance of subatomic particles. Among these fundamental building blocks, electrons play a pivotal role in shaping the very fabric of our existence. Understanding their enigmatic behavior is akin to unlocking the secrets of creation.
Quantum Mechanics: The Guiding Force
Electrons, unlike their macroscopic counterparts we can observe and interact with, exist in a realm governed by quantum mechanics. This peculiar set of rules defies our everyday experiences, where particles behave as both waves and particles simultaneously.
Wave-Particle Duality: A Paradoxical Nature
According to this enigmatic theory, electrons are not mere point-like objects but rather exist as wave-like entities with inherent probabilities of being found in specific locations. This wave-particle duality forms the cornerstone of electron behavior, allowing them to tunnel through energy barriers and exhibit quantum entanglement.
Orbital Theory: Mapping the Electron Landscape
The behavior of electrons in atoms is further illuminated by the concept of atomic orbitals. These orbitals are three-dimensional regions where electrons have the highest probability of being found. Each orbital possesses a unique shape and energy level, painting a vivid picture of electron distribution within the atom.
Pauli Exclusion Principle: The Unwritten Law of Electron Etiquette
As electrons dance within their atomic orbits, they adhere to a fundamental law: the Pauli exclusion principle. This principle states that no two electrons within an atom can occupy the same quantum state, ensuring a unique identity for each electron.
Implications for Chemistry and Biology:
The intricate behavior of electrons is not confined to the realm of physics but has profound implications for chemistry and biology. In chemical reactions, electrons are the key players in forming and breaking bonds between atoms, governing the formation of molecules that make up our world. Similarly, in biological systems, electron transfer is essential for energy metabolism, as exemplified by the vital role of cytochrome c in the electron transport chain.
Delving into the behavior of electrons is a captivating journey into the quantum realm, where the laws of physics unravel the mysteries of our universe. By unlocking the secrets of electron behavior, we gain a deeper understanding of the fundamental forces that shape our existence, from the interactions of atoms to the intricate dance of life.
The Anatomy of an Atom: A Microscopic Universe
Imagine you’re embarking on a journey to the heart of matter, where the building blocks of everything exist—atoms. Each atom is a tiny universe, harboring a wealth of secrets. At its core lies the nucleus, a dense, compact sphere consisting of positively charged protons and neutral neutrons. The nucleus holds the atom’s identity, carrying its atomic number, which defines the number of protons.
Surrounding the nucleus, like electrons orbiting a star, are negatively charged electrons. These electrons are arranged in discrete shells or energy levels, each with a specific capacity. The electrons in the outermost shell, known as valence electrons, are particularly crucial in determining an atom’s chemical behavior. The number of valence electrons dictates the atom’s tendency to bond with other atoms, forming molecules and creating the myriad of substances that make up our world.
So, the enigmatic atom, with its intricate structure and symphony of subatomic particles, is the cornerstone of all matter. Understanding the anatomy of atoms is akin to deciphering the language of the universe, unlocking the secrets of how it all works.
Valence Electrons: The Unsung Heroes of Chemical Bonding
In the bustling world of atoms, electrons play a pivotal role in determining the fate of chemical reactions. Among these electrons, a select group known as valence electrons holds the key to unlocking the secrets of chemical bonding.
Valence electrons are the electrons that reside in the outermost energy level of an atom. Their unique position makes them highly reactive and eager to interact with their surroundings. These electrons are the gatekeepers of chemical bonding, the force that unites atoms to form molecules.
When atoms come together, their valence electrons are the first to make contact. They form bonds by sharing or transferring electrons to achieve a stable electron configuration. This sharing or transfer of electrons results in the formation of chemical compounds with unique properties.
For example, sodium (Na) has one valence electron, while chlorine (Cl) has seven valence electrons. When sodium and chlorine interact, the sodium atom donates its valence electron to the chlorine atom, forming a stable compound called sodium chloride (NaCl).
Similarly, covalent bonds are formed when atoms share valence electrons. In this case, each atom contributes one or more valence electrons to form a shared pair of electrons. This shared pair of electrons creates a strong bond between the atoms, as both atoms have a vested interest in keeping the electrons close.
The number of valence electrons an atom has also determines its chemical reactivity. Atoms with a full outermost energy level (eight valence electrons) are generally less reactive because they have a stable electron configuration and are less likely to form bonds. On the other hand, atoms with incomplete outermost energy levels are more reactive because they are eager to gain or lose electrons to achieve stability.
Understanding the concept of valence electrons is fundamental to unraveling the intricacies of chemical reactions. These electrons act as the architects of chemical bonding, determining the properties and behavior of the compounds they create. By understanding the role of valence electrons, we gain a deeper appreciation for the complex world of atoms and molecules that shape our universe.
Cytochrome c: A Hemoprotein with a Remarkable Role
In the intricate world of biochemistry, there’s a fascinating protein that plays a pivotal role in our body’s energy production: cytochrome c. This hemoprotein is a small, soluble protein found in the mitochondria of eukaryotic cells.
The structure of cytochrome c is as intriguing as its function. It consists of a single polypeptide chain folded into a globular shape. The heart of this protein lies in heme, an iron-containing porphyrin complex. The iron ion is bound to the porphyrin ring by four nitrogen atoms, forming a planar structure. This unique arrangement allows cytochrome c to interact with electrons efficiently.
Fun Fact: Cytochrome c was one of the first proteins to have its crystal structure solved, providing valuable insights into the structure-function relationship of proteins.
The Electron Transport Chain: A Vital Process Unveiled
In the intricate world of cellular respiration, a crucial process unfolds within the mitochondria: oxidative phosphorylation. This phenomenon powers our bodies by generating the energy currency known as ATP (adenosine triphosphate). And at the heart of this process lies the electron transport chain.
Imagine a series of membrane-bound proteins, each strategically positioned in the mitochondrial membrane. These proteins, like tiny cogs in a molecular machine, work in concert to facilitate the transfer of electrons. As electrons flow through this chain, they release energy that is harnessed to pump protons across the membrane, creating a proton gradient.
The proton gradient, in turn, drives the final step of ATP synthesis through a protein channel known as ATP synthase. This remarkable process ensures that energy is efficiently harnessed and stored for cellular needs.
Cytochrome c: The Electron Shuttle in Cellular Respiration
In the intricate world of cellular biology, electrons play a vital role in fueling the energy processes that sustain life. Among the key players in this electron dance is cytochrome c, a fascinating protein that serves as an electron shuttle in the electron transport chain.
The Electron Transport Chain: Energy from Electron Flow
The electron transport chain is a series of membrane-bound proteins embedded in the inner mitochondrial membrane. It’s like a molecular relay race where electrons are passed from one protein to another, energy is released, and ATP, the energy currency of cells, is produced.
Cytochrome c’s Orchestrated Electron Transfer
Cytochrome c is a small, water-soluble protein that resides in the intermembrane space of mitochondria. Its structure comprises a single heme group, an iron-containing molecule that can reversibly bind electrons.
Cytochrome c acts as an electron shuttle between two protein complexes in the electron transport chain:
- Complex III (Cytochrome bc1 Complex): Receives electrons from a high-energy molecule called ubiquinone.
- Complex IV (Cytochrome c Oxidase): The final electron acceptor in the chain, passing electrons to oxygen to form water.
The Electron Transfer Dance
Cytochrome c binds to both complex III and complex IV, enabling the seamless transfer of electrons between these two complexes. The reduction of oxidized cytochrome c (Fe3+) to its reduced form (Fe2+) at complex III provides the energy to pump protons across the mitochondrial membrane, contributing to the proton gradient that drives ATP synthesis.
Electron Transfer: A Vital Cog in the Energy Machinery
Understanding electron transfer in cytochrome c is crucial for comprehending cellular respiration and energy metabolism. The efficient operation of this protein ensures that the body has a steady supply of ATP, the fuel that powers cellular processes and sustains life.
In summary, cytochrome c is a remarkable electron carrier that orchestrates the electron flow in the electron transport chain, enabling cells to harness energy and thrive. Its role as an electron shuttle is essential for maintaining cellular respiration and energy balance, making it a fascinating player in the intricate symphony of life.
Hemoglobin and Myoglobin: Electron Carriers of a Similar Kind
In the realm of biology, the transfer of electrons plays a crucial role in the efficient functioning of cells. Cytochrome c, a protein found in the electron transport chain, plays a pivotal role in this process. However, it’s not alone; there are other electron-carrying proteins that share similarities with cytochrome c, namely hemoglobin and myoglobin.
All three proteins possess a similar structure that enables them to bind and transport electrons. They consist of heme groups, which are porphyrin rings containing an iron ion. This iron ion is what gives these proteins their ability to bind and carry electrons.
Hemoglobin, found in red blood cells, is responsible for transporting oxygen throughout the body. It binds to oxygen molecules through its heme groups and delivers them to cells that need them. Myoglobin, found in muscle cells, also binds to oxygen but serves a different purpose. It stores oxygen for muscle cells to use during periods of high demand, such as during exercise.
Despite their different functions, cytochrome c, hemoglobin, and myoglobin all share the common role of electron carriers. They facilitate the transfer of electrons in various cellular processes, ensuring the proper functioning of the body. By understanding the role of these proteins in electron transfer, we gain a deeper appreciation for the intricate workings of cells and the fundamental processes that sustain life.
The Significance of Electron Transfer in Cells: Unraveling the Secrets of Energy Metabolism
Delving into the intricate world of cells, we stumble upon a remarkable dance of electrons, orchestrating the vital symphony of life. Electron transfer is a pivotal process that underpins the very essence of cellular respiration and energy metabolism.
Cellular respiration fuels our cells with the energy they need to thrive. It’s a intricate process that harnesses the chemical energy stored in food and converts it into a currency that our bodies can use: ATP (adenosine triphosphate).
At the heart of cellular respiration lies a series of membrane-bound proteins known as the electron transport chain. Electrons, liberated from food molecules, embark on a rollercoaster ride through this chain, passing from one protein complex to another.
Cytochrome c is a crucial player in this electron transfer ballet. It’s a hemoprotein, meaning it contains an iron-porphyrin complex. This complex acts as a relay station, accepting electrons from complex III and handing them off to complex IV.
As electrons flow through the electron transport chain, they lose energy. This lost energy is harnessed to pump protons across the membrane. The buildup of protons creates a gradient, driving the production of ATP through a process called oxidative phosphorylation.
Understanding electron transfer in cytochrome c is like unlocking a secret code to cellular respiration. It’s a key to deciphering how our bodies convert food into the life-sustaining energy that fuels every beat of our hearts and every breath we take.