Understanding Covalent Bonds: Factors Influencing Bond Strength And Polarity
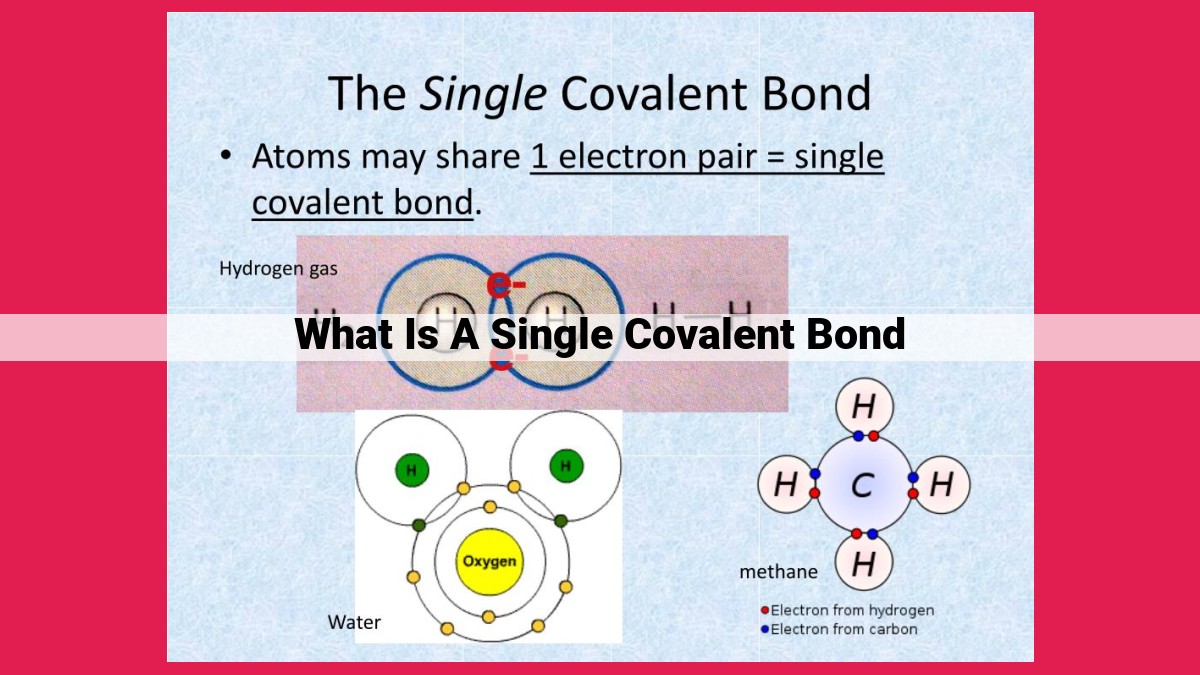
A single covalent bond forms when two atoms share a pair of electrons, resulting in a strong chemical bond. The electronegativity difference between the atoms determines the polarity of the bond, with a large difference leading to ionic bonds. Bond strength is influenced by bond order (the number of shared electron pairs) and bond length (the distance between the atoms). Bond length is determined by the atomic radii and the hybridization of the atoms, which involves mixing atomic orbitals to form new hybrid orbitals that optimize bond formation.
Definition of a Single Covalent Bond
- Explain what a single covalent bond is and how it forms.
- Discuss the concept of electron sharing.
Single Covalent Bonds: Understanding the Dance of Shared Electrons
In the realm of chemistry, bonds are the ties that bind atoms together, forming the building blocks of molecules and the substances that make up our world. Among the different types of bonds, the single covalent bond stands out as a fundamental force in molecular bonding. Let’s embark on a journey to unravel the secrets of single covalent bonds.
Imagine two neighboring atoms, each with an unpaired electron yearning to find a dance partner. As these atoms come close, their atomic orbitals, hazy clouds of electrons surrounding each nucleus, start to overlap. Like two perfectly matched puzzle pieces, these orbitals merge together, creating a molecular orbital. Within this molecular orbital, the unpaired electrons from each atom entwine, forming a covalent bond.
The formation of a single covalent bond is a delicate dance of electron sharing. The two atoms contribute one electron each to the molecular orbital, effectively sharing them to form a bond. This shared pair of electrons becomes the glue that holds the atoms together, creating a stable molecular entity.
The strength of this bond is determined by the electronegativity of the atoms involved. Electronegativity is a measure of an atom’s ability to attract electrons towards itself. When two atoms of similar electronegativity bond, they form a nonpolar covalent bond. In such a bond, the electrons are evenly shared between the atoms. However, when atoms with significantly different electronegativity bond, they form a polar covalent bond. In this case, the more electronegative atom attracts the shared electrons more strongly, creating a slight imbalance in charge distribution.
Electronegativity Difference and Its Impact on Covalent Bonding
In the realm of covalent bonding, where atoms unite not by force but by the allure of shared electrons, the electronegativity difference plays a crucial role in determining the bond’s character. Electronegativity, a measure of an atom’s electron-pulling power, creates an imbalance when two atoms with different electronegativities come together.
This electronegativity difference can lead to two distinct types of covalent bonds: polar covalent bonds and ionic bonds. In a polar covalent bond, the electrons are not shared equally between the atoms. One atom, with a greater electronegativity, exerts a stronger pull on the electrons, creating a slight imbalance in the distribution. This results in a partial positive charge on one atom and a partial negative charge on the other.
In contrast, an ionic bond forms when the electronegativity difference is significant enough to cause the complete transfer of an electron from one atom to another. This complete transfer results in the formation of two oppositely charged ions, held together by the electrostatic force.
The electronegativity difference not only determines the polarity of covalent bonds but also provides insights into the bond’s strength. A larger electronegativity difference indicates a greater imbalance in the electron distribution, leading to a more polar covalent bond. This increased polarity can weaken the bond strength as the opposing charges repel each other. Conversely, a smaller electronegativity difference results in a more equal sharing of electrons, creating a stronger covalent bond.
Understanding the electronegativity difference is key to comprehending the diverse nature of covalent bonding. It governs the polarity of the bond, which in turn influences the bond strength and ultimately shapes the properties of molecules and materials.
Delving into the Strength of Chemical Bonds
In the intricate dance of atoms, covalent bonds emerge as the fundamental players, holding molecules together with a remarkable display of strength. But what exactly determines how strong these bonds are? Let’s unveil the secrets behind bond strength.
Bond Strength: The Essence of Stability
Picture a covalent bond as a tug-of-war between the shared electrons, each atom pulling in its favor. Bond strength quantifies this tug-of-war’s intensity, indicating how tightly the electrons are held between the atoms.
Bond Order: The Key to Strong Bonds
Enter bond order, the concept that dictates the strength of the bond. It represents the number of shared electron pairs between the atoms. The more shared pairs, the greater the bond order and the stronger the bond.
Bond Length: The Inverse Relationship
Curiously, bond strength and bond length exhibit an inverse relationship. As bond length increases, the strength of the bond decreases. Imagine a spring stretched between atoms; the farther apart the atoms, the weaker the spring’s pull.
The Intricate Dance of Atoms
Understanding bond strength is essential for comprehending the very fabric of molecules. Strong bonds hold atoms firmly together, forming stable structures, while weaker bonds allow for flexibility and adaptability in molecular shape and function.
In summary, bond strength serves as a vital measure of the stability of covalent bonds. It depends on the bond order and bond length, offering insights into the intricate dance of atoms that shape the world around us.
Bond Length: A Tale of Atomic Radii and Bond Order
In the realm of covalent bonding, the length of a bond is a crucial parameter that governs the molecule’s structure and properties. Enter bond length, the distance between two bonded atoms’ nuclei.
Atomic Radius: The Measure of an Atom’s Reach
Picture this: atoms are like spherical balls, their size determined by the atomic radius. This radius represents the extent of an atom’s electron cloud, the region where its electrons reside. The atomic radius, a fundamental property, varies from element to element due to differences in the number and arrangement of electrons.
Covalent Radius: When Atoms Share Space
When atoms join hands in a covalent bond, they share electrons to form a molecular bond. The covalent radius is the effective radius of an atom when it’s involved in a covalent bond. This radius is usually smaller than the atomic radius because the shared electrons pull the nuclei closer together.
Bond Order: The Dance of Electron Pairs
The bond order unveils the number of electron pairs shared between two atoms. A single bond, the simplest form of covalent bond, involves one shared electron pair. Double and triple bonds, more prevalent, share two and three electron pairs, respectively.
The Bond Length Equation: A Balancing Act
Now, let’s unveil the equation that links bond length, atomic radii, and bond order:
Bond Length = (Covalent Radius of Atom 1 + Covalent Radius of Atom 2) / Bond Order
Like a delicate balance, bond length is a combination of the two atoms’ covalent radii and the number of electron pairs they share. The higher the bond order, the shorter the bond length because the shared electrons hold the atoms closer together.
Impact on Molecular Geometry
Bond length plays a pivotal role in shaping molecular geometry. Shorter bonds allow for closer atomic packing, leading to more compact molecular structures. Longer bonds, on the other hand, result in more elongated structures.
Bond length is a window into the molecular world, revealing the dance between atoms as they come together to form covalent bonds. Understanding bond length illuminates the structure and properties of countless molecules that make up our world, from the simple to the complex.
Understanding Bond Order: The Key to Covalent Bonding
In the captivating realm of chemistry, covalent bonding plays a central role in defining the characteristics and behavior of countless molecules. Among its key concepts, bond order stands tall as a crucial factor in determining the strength and nature of covalent bonds.
Defining Bond Order
Bond order, in essence, measures the number of shared electron pairs between two atoms. Covalent bonds arise when atoms share electrons to achieve a more stable electronic configuration, similar to two people sharing a seat for comfort. The higher the bond order, the stronger the covalent bond and the more stable the resulting molecule.
Multiple Covalent Bonds: The Case of Double Bonds
When atoms share two pairs of electrons, they form a double bond. This increased electron density between the atoms leads to an even stronger bond. Double bonds are prevalent in many organic molecules, contributing to their unique properties. For instance, double bonds in carbon-carbon chains give rise to the flexibility and strength of plastics.
Bond Order and Molecular Structure
Bond order also influences the molecular structure and geometry of molecules. Double bonds, for example, introduce a rigid shape, with the participating atoms arranged in a linear fashion. This shape stems from the overlap of two pairs of atomic orbitals, creating a region of high electron density between the atoms.
Bond order emerges as a pivotal concept in understanding the nature of covalent bonding. It provides insights into bond strength, molecular structure, and the behavior of countless molecules. By unraveling the intricacies of bond order, chemists gain a deeper appreciation for the fascinating world of molecular bonding.
Hybridization: Unlocking the Secrets of Molecular Geometry
In the captivating world of chemistry, hybridization stands as a pivotal concept that governs how atoms form bonds and shape molecules. Picture this: atoms, like tiny building blocks, possess unpaired electrons eager to connect. But not all connections are created equal. Hybridization, like a skilled architect, orchestrates the blend of atomic orbitals to create new hybrid orbitals with unique geometries and properties.
Navigating the Hybrid Landscape
The types of hybrid orbitals depend on the number and type of atomic orbitals involved in the hybridization. The most common types include:
- sp³ hybrid orbitals: Formed by the mixing of one s orbital and three p orbitals, resulting in four equivalent hybrid orbitals that point towards the corners of a tetrahedron.
- sp² hybrid orbitals: A fusion of one s orbital and two p orbitals, creating three hybrid orbitals arranged in a trigonal planar geometry.
- sp hybrid orbitals: A combination of one s orbital and one p orbital, giving rise to two linear hybrid orbitals.
The Architectural Blueprint of Molecules
These hybrid orbitals serve as the foundation for molecular geometry. The spatial arrangement of these hybrid orbitals dictates the shape of the molecule. For instance, four sp³ hybrid orbitals give rise to a tetrahedral molecular geometry, while three sp² hybrid orbitals result in a trigonal planar geometry.
Hybridization in Action
Hybridization plays a crucial role in countless aspects of chemistry, including:
- Bond formation: The hybrid orbitals of different atoms overlap, forming strong covalent bonds.
- Molecular polarity: The electronegativity difference between bonded atoms influences the polarity of the molecule.
- Reactivity: The hybridization of atoms can affect the reactivity of molecules in chemical reactions.
Unveiling the Magic of Hybridization
Hybridization is not just a complex scientific concept but a testament to the intricate symphony of nature. It underpins the diverse structures and properties of molecules that orchestrate the wonders of life. From the captivating shapes of snowflakes to the intricate workings of DNA, hybridization reveals the hidden language of matter.