Conservation In Chemical Reactions: Unraveling The Principle Of Invariance
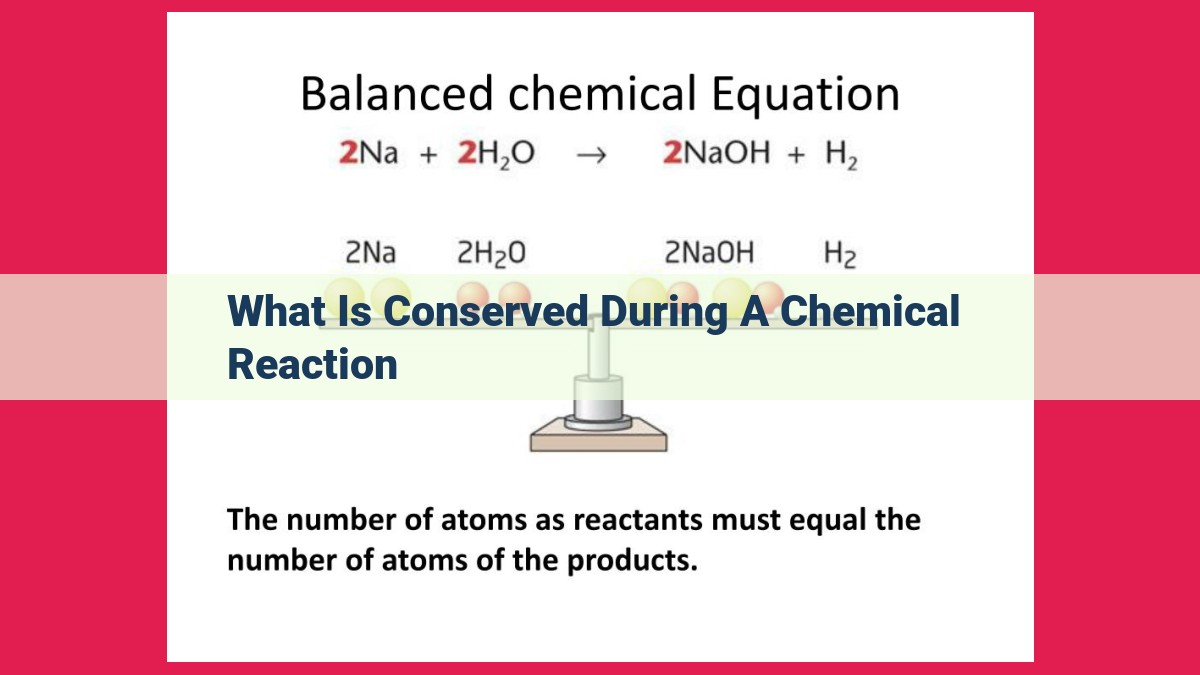
During chemical reactions, certain quantities remain constant, showcasing the principle of conservation. Mass, atoms, charge, energy, momentum, angular momentum, and entropy are all conserved. This means that within a closed system, the total mass, number of atoms of each element, overall charge, and total energy remain unaltered throughout the reaction. Understanding conservation aids in predicting reaction outcomes, balancing chemical equations, and comprehending the fundamental principles governing chemical transformations.
The Fundamentals of Conservation During Chemical Reactions
Imagine you have a bag filled with marbles of different colors. When you pour these marbles into another bag, something fascinating happens: the total number of marbles remains the same. This principle of conservation applies to more than just marbles; it’s a fundamental law that governs the realm of chemical reactions.
Chemical reactions are like magic tricks that transform one set of substances into another. But amidst all the excitement, there’s a hidden orderliness. Just like you can’t make or lose marbles in our marble analogy, you can’t create or destroy atoms or matter during chemical reactions. This is known as the conservation of mass.
Beyond mass, atoms themselves are conserved. Even as they rearrange and combine to form new molecules, the total number of each type of atom remains constant. This is a testament to the identity and integrity of every atom.
Another important aspect of conservation is charge. Charge, like magic, is a property that can be either positive or negative. In chemical reactions, the total charge of all the atoms and molecules involved stays the same. It’s like a balancing act between positive and negative charges, ensuring that the overall electrical neutrality is preserved.
And now, let’s talk energy. Like money in a bank account, energy can’t be created or destroyed, but it can be transformed. In chemical reactions, energy is often transferred or released as heat or light. But no matter what form it takes, the total amount of energy remains constant. This is known as the conservation of energy.
Finally, even the subtlest changes come under the umbrella of conservation. Entropy, a measure of disorder, increases in most chemical reactions. This is because reactions tend to distribute energy and molecules more evenly, leading to a higher state of disorder or randomness.
In conclusion, chemical reactions are intricate dances governed by the unwavering laws of conservation. From the conservation of atoms to the conservation of energy, these principles ensure that the essence of matter and charge remains untouched. It’s like a grand symphony, where each element and molecule plays its designated role, preserving the delicate balance of the chemical realm.
The Fundamentals of Conservation During Chemical Reactions
Embark on a captivating journey into the fascinating world of chemical reactions, where the enigmatic concept of conservation reigns supreme. Imagine witnessing the transformative power of chemistry, where substances are altered and new creations emerge. Throughout this extraordinary process, certain fundamental principles remain steadfast, ensuring that the delicate balance of the universe is preserved.
As we delve into the intricacies of chemical reactions, we’ll unravel the secrets of conservation and discover how it governs the transformation of matter and energy. From the conservation of mass to the preservation of energy, these principles provide a roadmap to understanding the intricate dance of atoms and molecules within chemical reactions.
Section 2: The Enigmatic Conservation of Mass
In the realm of chemical reactions, the concept of mass takes center stage, playing a pivotal role in understanding the transformations that occur. Mass, an intrinsic property of matter, represents the quantity of material it contains. Amazingly, during chemical reactions, the total mass remains constant. This fundamental principle, known as the conservation of mass, ensures that the universe’s mass remains unchanged.
Just as a skilled accountant meticulously balances the books, the universe maintains a delicate equilibrium in terms of mass. During chemical reactions, atoms are neither created nor destroyed, but merely rearranged to form new substances. The total mass of the reactants (the starting materials) is identical to the total mass of the products (the resulting substances).
Mass, however, is not the only aspect that remains constant. The relationship between mass, weight, density, and volume also comes into play. Weight, often used interchangeably with mass, actually measures the force of gravity acting on an object. Density, on the other hand, measures the compactness of matter, while volume measures the space it occupies.
While mass is an invariant quantity during chemical reactions, these other properties may change. For instance, a solid reactant may have a higher density than its gaseous product, indicating a reduction in compactness. Similarly, the weight of a reactant may vary depending on the gravitational field it experiences.
Understanding the conservation of mass is not only critical for balancing chemical equations but also for solving stoichiometry problems. It allows us to predict the amount of reactants and products involved in a reaction and to determine the limiting reactant that dictates the extent of the reaction.
Section 3: Conservation of Atoms
At the very heart of every chemical reaction lies a fundamental principle known as the conservation of atoms. This principle asserts that within the intricate dance of chemical reactions, the number of atoms of each element remains constant. It’s as if there’s an invisible accountant watching over every reaction, ensuring that the number of atoms of each element never wavers.
Atoms: The Building Blocks of Matter
Before we delve deeper into the conservation of atoms, let’s first meet the smallest building blocks of matter: atoms. These tiny particles are composed of a dense center called a nucleus, surrounded by a cloud of whizzing electrons. Each atom is unique, characterized by its atomic number, which tells us the number of protons in its nucleus.
Molecules: When Atoms Team Up
Atoms rarely travel alone. They often join forces to form molecules, which are two or more atoms chemically bonded together. Take water, for example. Its molecules consist of two hydrogen atoms and one oxygen atom. This trio forms a molecule of H₂O, bringing life to our planet.
Elements and Compounds: Cousins of the Periodic Table
The elements are the basic units of matter, each with a different atomic number. They’re the building blocks of the periodic table, arranged in a dance of increasing atomic numbers. Compounds, on the other hand, are substances made up of two or more different elements chemically combined. For instance, the salt you sprinkle on your fries is a compound called sodium chloride, composed of sodium atoms and chlorine atoms.
Conservation of Atoms in Action
Now, let’s return to the stage where atoms perform their dance of conservation. During a chemical reaction, atoms may rearrange themselves, forming new substances. But throughout this transformation, the total number of atoms of each element remains unchanged. It’s as if each atom has its own unique serial number, and they can’t simply vanish or appear out of thin air.
For example, when iron rusts, the iron atoms in the metal combine with oxygen atoms from the air to form a new compound called iron oxide. But don’t be fooled by its new appearance. Count the number of iron atoms and oxygen atoms in iron oxide, and you’ll find that it’s the same as the number of atoms you started with.
The conservation of atoms is a cornerstone of chemistry, guiding our understanding of chemical reactions. It’s a testament to the fundamental order within the seemingly chaotic world of matter.
Section 4: Embracing the Unchanging Dance of Charge
In the realm of atomic interactions, charge takes center stage as a fundamental property that governs the behavior of matter. Charge, like an unseen puppeteer, orchestrates the movements of electrons and protons, shaping the very essence of chemical reactions.
Electric fields, magnetic fields, and voltage all pay homage to the power of charge. Electric fields exert their influence through the interaction of charged particles, guiding their trajectories like invisible magnets. Magnetic fields, too, arise from the motion of charged particles, creating swirling forces that influence the paths of their fellow travelers. Voltage, the measure of electrical potential, reflects the energy that drives charged particles to move.
In the intricate choreography of chemical reactions, charge plays a pivotal role. As atoms and molecules undergo transformations, the total charge of the system remains invariably constant. This fundamental principle, known as the conservation of charge, ensures that the number of positive charges always balances the number of negative charges.
While atoms may gain or lose electrons, altering their charge state, the overall charge of the system remains unaltered. It’s as if the universe maintains a cosmic scoreboard, keeping a meticulous tally of every electron and proton, ensuring that the equilibrium of charge is never disrupted.
This conservation of charge serves as a guiding star for chemists, helping them unravel the complexities of chemical reactions. By understanding how charge flows and transforms, scientists can predict the outcomes of reactions and design new materials with tailored properties. It’s a testament to the power of understanding the fundamental principles that govern our world.
Section 5: Conservation of Energy
In the realm of chemical reactions, energy plays a crucial role. It is the driving force that initiates, sustains, and ultimately determines the outcome of these transformations. So, what is energy, and how does it behave within the confines of a chemical reaction?
Defining Energy
Energy, in its many guises, is the capacity to perform work or generate change. It manifests itself in various forms, including:
- Kinetic Energy: The energy an object possesses due to its motion.
- Potential Energy: The energy stored within an object due to its position or condition.
- Thermal Energy: The energy associated with the random motion of particles.
- Chemical Energy: The energy stored within the bonds between atoms and molecules.
Energy Conservation in Chemical Reactions
During a chemical reaction, energy is not created or destroyed, but rather transformed from one form to another. This principle, known as conservation of energy, ensures that the total energy of the system remains constant throughout the reaction.
When a chemical bond breaks, energy is absorbed, and this absorbed energy is released when a new bond is formed. The net change in energy during a reaction is what determines whether it is exothermic (energy released) or endothermic (energy absorbed).
For example, in the combustion of methane, the chemical energy stored in the methane molecules is converted into thermal energy (heat) and light energy (in the form of flames). This chemical reaction is exothermic, as the total energy of the products (heat and light) is higher than that of the reactants (methane and oxygen).
In contrast, in the photosynthesis reaction, light energy is absorbed by plants and used to convert carbon dioxide and water into glucose and oxygen. This reaction is endothermic, as the total energy of the products (glucose and oxygen) is lower than that of the reactants (carbon dioxide and water).
Regardless of whether a reaction is exothermic or endothermic, the total energy of the system remains constant. This fundamental principle of conservation of energy is a cornerstone of chemical reactions and underpins our understanding of chemical transformations.
Section 6: Conservation of Momentum
Momentum, a fundamental property of moving objects, is defined as the product of its mass and velocity. Inertia, the tendency of an object to resist changes in motion, is directly related to its mass. As velocity measures the speed and direction of movement, acceleration quantifies the rate at which velocity changes.
In the fascinating realm of chemical reactions, momentum is just as crucial as mass, atoms, and energy. Conservation of momentum dictates that the total momentum of a closed system, meaning one where no external forces act, remains constant throughout the reaction.
Imagine two billiard balls colliding on a frictionless table. As one ball strikes the other, it imparts momentum to it, causing the second ball to move. Simultaneously, the first ball experiences an equal but opposite change in momentum, leading to its movement in the opposite direction. The total momentum of the system, the sum of their individual momenta, remains unchanged throughout the collision.
Similarly, in chemical reactions, atoms and molecules exchange momentum as they rearrange and recombine. Consider the combustion of methane, where methane molecules react with oxygen molecules to produce carbon dioxide and water molecules. As the methane and oxygen molecules approach each other, they possess a certain amount of momentum. Upon collision, they exchange momentum, resulting in the formation of new molecules with different velocities. However, the total momentum of the system, the collective momentum of all atoms and molecules involved, remains constant from the start to the end of the reaction.
Conservation of momentum is a cornerstone of chemical kinetics, helping scientists understand the dynamics of chemical reactions and predict the trajectories of reactants and products. By unraveling the intricate dance of momentum exchange, chemists gain valuable insights into the behavior of matter at the molecular level.
Section 7: Conservation of Angular Momentum
Angular momentum, a fundamental quantity in physics, describes the rotational motion of objects. It’s like a twirling figure skater who spins faster with their arms tucked in to conserve their angular momentum. In chemical reactions, too, this principle holds true.
Angular momentum is determined by two key factors: torque and moment of inertia. Torque, the twisting force, acts like a push or pull that sets objects in motion. Moment of inertia, on the other hand, is a measure of how difficult it is to alter an object’s rotational motion; think of a heavy flywheel that takes more effort to spin up.
In chemical reactions, angular momentum is conserved, meaning the total angular momentum of the reactants equals the total angular momentum of the products. This conservation principle ensures that as molecules rearrange and rotate, their overall angular momentum remains unchanged. It’s as if the reaction is a dance where the total spin stays constant throughout each intricate move.
Consider a simplified chemical reaction between two molecules, A and B. Imagine molecule A spinning clockwise and molecule B spinning counterclockwise. When they collide, they exchange energy and momentum, but the total angular momentum is conserved. The resulting molecules will rotate at different speeds, but the overall spin remains the same.
This principle of conservation of angular momentum plays a crucial role in understanding the dynamics of chemical reactions, particularly those involving rotational motion. It provides a powerful tool for scientists to analyze and predict the outcomes of these reactions, unlocking insights into the complex world of molecular interactions.
Change in Entropy: The Measure of Disorder in Chemical Reactions
When delving into the realm of chemistry, we often encounter the concept of entropy, a fascinating property that governs the level of disorder within a system. Entropy is the measure of the energy that is present within a system and not available for work. It is a measure of the randomness or disorder of a system, and it tells us how much a system tends to spontaneously change towards a more random state.
In the context of chemical reactions, entropy plays a crucial role in determining the reaction’s direction and spontaneity. As a general rule, chemical reactions tend to favor an increase in entropy. This means that reactions that result in a greater level of disorder will be more likely to occur spontaneously.
For example, when a solid dissolves in a liquid, it results in an increase in disorder as the molecules of the solid become more spread out throughout the liquid. This increased disorder leads to a positive change in entropy, making the dissolution process spontaneous.
On the other hand, some reactions may result in a decrease in entropy. For instance, when a gas condenses to a liquid, the molecules become more closely packed, leading to a decrease in disorder. This decrease in entropy results in a negative change in entropy, making the condensation process non-spontaneous.
The change in entropy during a chemical reaction can be calculated using the following equation:
ΔS = Sfinal - Sinitial
where ΔS represents change in entropy, Sfinal is the entropy of the final state, and Sinitial is the entropy of the initial state. A positive ΔS indicates an increase in disorder, while a negative ΔS indicates a decrease in disorder.
Understanding the concept of entropy is crucial for comprehending the spontaneity of chemical reactions. It provides valuable insights into the direction and orderliness of chemical processes, helping us predict the behavior of matter under various conditions.