Comprehensive Guide To Mrna Processing: Splicing, Capping, Polyadenylation, And Beyond
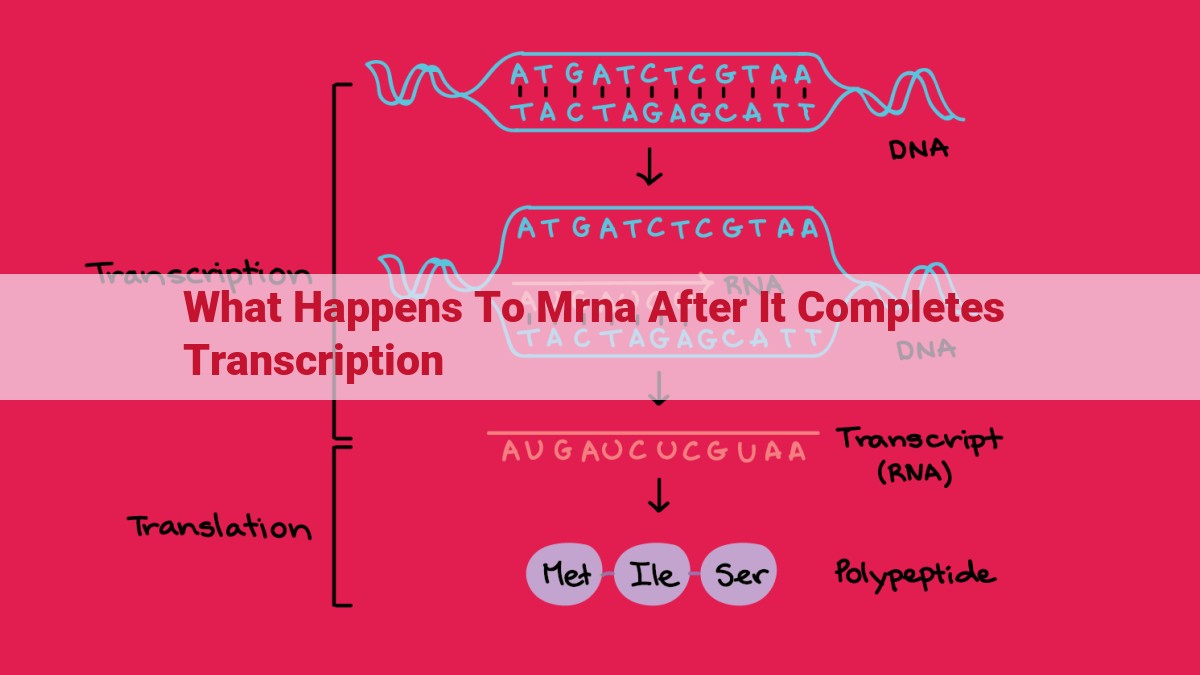
After transcription, mRNA undergoes RNA splicing to remove non-coding introns, mRNA capping to protect the 5′ end with a modified guanine, and mRNA polyadenylation to add a poly(A) tail to the 3′ end. These modifications enhance mRNA stability, translation efficiency, and nuclear export. mRNA is then transported to the cytoplasm for translation, where ribosomes decode its genetic code to synthesize proteins. Finally, mRNA is degraded by RNAse enzymes to regulate gene expression and cellular processes.
RNA Splicing: Editing the mRNA Blueprint
Journey to a Precise Genetic Blueprint
In the realm of gene expression, RNA splicing plays a pivotal role in ensuring that only the essential genetic information reaches its destination. Like a master editor, it meticulously removes non-coding introns – the unwanted segments of RNA – and seamlessly joins the coding exons, creating a precise blueprint for protein synthesis.
The Spliceosome: A Molecular Matchmaker
Orchestrating this delicate splicing process is a molecular virtuoso known as the spliceosome. Comprised of a multitude of small nuclear ribonucleoprotein complexes (snRNPs), the spliceosome acts as a microscopic matchmaker, identifying the precise boundaries between introns and exons. With precision and elegance, it cuts away the introns, leaving behind a refined mRNA molecule that contains only the genetic code for protein synthesis.
Unveiling the Importance of RNA Splicing
The remarkable process of RNA splicing has far-reaching implications for cellular function. By removing introns, it ensures that only the necessary genetic information is translated into proteins, preventing the production of non-functional or harmful proteins. Moreover, alternative splicing, where different exons are joined together, allows for the production of diverse protein isoforms from a single gene, expanding the repertoire of cellular functions.
mRNA Capping: Protecting the Message
In the intricate tapestry of cellular life, mRNA (messenger RNA) plays a pivotal role in translating the genetic code into functional proteins. However, this delicate molecule is inherently fragile and vulnerable to degradation. To safeguard its integrity, a crucial process known as mRNA capping has evolved. It’s like putting on a protective shield, ensuring the message reaches its destination intact.
Unveiling the mRNA Capping Mechanism
At the very beginning of the mRNA molecule, an extraordinary chemical transformation occurs. A modified guanine nucleotide, adorned with an unusual methyl group, is meticulously attached to the 5′ end. This seemingly small addition packs a powerful punch in the world of mRNA stability and function.
Benefits of mRNA Capping
The benefits of mRNA capping are as multifaceted as they are essential:
Enhanced Stability: The protective cap acts as a fortress, shielding the mRNA from enzymatic degradation. Without it, mRNA would be vulnerable to premature destruction, hindering protein synthesis.
Ribosome Recognition: The modified guanine nucleotide serves as a beacon, guiding ribosomes to the correct starting point on the mRNA. This ensures accurate translation of the genetic code.
Protection from Exonucleases: mRNA molecules are susceptible to exonucleases, enzymes that nibble away at their edges. The cap provides a physical barrier, preventing these molecular predators from devouring the mRNA.
mRNA Polyadenylation: The Key to mRNA Stability and Export
As the blueprint for protein synthesis, mRNA plays a crucial role in gene expression. However, before mRNA can fulfill its destiny, it undergoes a crucial modification known as polyadenylation, the addition of a poly(A) tail to its 3′ end. This seemingly small modification has a profound impact on mRNA’s stability, ribosome recruitment, and nuclear export.
Polyadenylation is a complex process orchestrated by a protein complex called the cleavage and polyadenylation specificity factor (CPSF). CPSF recognizes a specific sequence in the mRNA, the polyadenylation signal, and cleaves the RNA at this site. This cleavage triggers the addition of a poly(A) tail of approximately 250 nucleotides by an enzyme called poly(A) polymerase.
The poly(A) tail serves several critical functions:
-
Increased Stability: Polyadenylation significantly increases mRNA stability by protecting it from degradation by exonucleases, enzymes that degrade RNA from their ends. The poly(A) tail acts as a protective cap, shielding the mRNA from enzymatic attack.
-
Ribosome Recruitment: The poly(A) tail plays a pivotal role in ribosome recruitment. It binds to specific poly(A)-binding proteins (PABPs), which in turn recruit ribosomes to the mRNA. This binding ensures efficient translation, the conversion of mRNA into protein.
-
Nuclear Export: Polyadenylation is also essential for the export of mRNA from the nucleus to the cytoplasm, where translation occurs. The poly(A) tail signals to nuclear export factors, which bind to the mRNA and facilitate its transport out of the nucleus.
In summary, mRNA polyadenylation is a crucial post-transcriptional modification that ensures the stability, ribosome recruitment, and nuclear export of mRNA. By protecting the mRNA from degradation, promoting ribosome binding, and facilitating nuclear export, polyadenylation plays a fundamental role in gene expression and protein synthesis.
mRNA Transport: The Journey from the Nuclear Vault to the Cytoplasmic Factory
Once the mRNA blueprint is finalized through splicing, capping, and polyadenylation, it embarks on a crucial journey from its birthplace in the nucleus to its destined destination in the cytoplasm. This carefully orchestrated transport process ensures that the genetic message contained within the mRNA reaches the ribosomes, the protein-making machinery of the cell.
A Molecular Escort: RNA-Binding Proteins and Transport Factors
Guiding this mRNA voyage are essential molecular chaperones known as RNA-binding proteins and transport factors. These proteins form a protective escort around the mRNA, shielding it from degradation and guiding it through the nuclear membrane and into the cytoplasm.
Through the Nuclear Gates: The Nuclear Pore Complex
The nuclear envelope, the fortress surrounding the nucleus, is not impenetrable. It contains specialized gateways called nuclear pore complexes. These pores, like intricate sieves, allow certain molecules, including mRNA, to pass through while blocking others. With the help of its molecular escorts, mRNA successfully navigates these intricate channels, traversing the nuclear membrane.
Into the Cytoplasmic Realm: The Road to Protein Production
Once in the cytoplasm, the mRNA is ready to fulfill its destiny. It seeks out the ribosomes, the cellular machines responsible for synthesizing proteins. RNA-binding proteins and transport factors continue to escort the mRNA, ensuring its safe delivery to these protein factories.
The Importance of mRNA Transport
Efficient mRNA transport is indispensable for cellular function. It ensures that proteins are produced where and when they are needed. Dysruptions in mRNA transport can lead to a host of cellular abnormalities, including developmental defects and diseases such as cancer.
By understanding the intricate tale of mRNA transport, we gain a deeper appreciation for the sophisticated machinery that underpins cellular life. The journey from the nucleus to the cytoplasm is a testament to the remarkable complexity and precision of the cellular processes that govern our very existence.
mRNA Translation: Decoding the Genetic Code
In the symphony of life, the message encoded in DNA is first transcribed into an RNA molecule, called messenger RNA (mRNA). This mRNA molecule carries the genetic blueprint out of the nucleus, where it embarks on a remarkable journey to translate this message into the proteins that orchestrate all aspects of cellular life. This process, known as mRNA translation, represents the final step in the central dogma of molecular biology.
The Ribosome: The Protein Synthesis Machine:
Translation is carried out by cellular machinery called ribosomes. These intricate structures are composed of ribosomal RNA (rRNA) and proteins and function as the central hub for protein synthesis. Ribosomes are shaped like small factories, complete with docking stations for mRNA and transfer RNA (tRNA) molecules.
The Genetic Code and tRNA:
The sequence of nucleotides in mRNA represents a series of three-nucleotide codons. Each codon corresponds to a specific amino acid. tRNA molecules are adapter molecules that recognize and bind to the codons on mRNA. Each tRNA carries a specific amino acid on its other end.
Step-by-Step Translation:
Translation occurs in several steps:
- Initiation: The ribosome assembles at a start codon (AUG) on the mRNA molecule. A specific tRNA molecule carrying the amino acid methionine binds to the start codon.
- Elongation: The ribosome moves along the mRNA, one codon at a time, as tRNA molecules bring in the corresponding amino acids. The ribosome catalyzes the formation of peptide bonds between these amino acids, building a growing polypeptide chain.
- Termination: When a stop codon is reached on the mRNA, a release factor binds to the ribosome. This causes the ribosome to release the finished polypeptide chain and disassemble.
The Importance of mRNA Translation:
mRNA translation is crucial for life. It enables cells to produce the proteins necessary for carrying out their specific functions, such as metabolism, cell division, and communication. Errors in translation can lead to the production of abnormal or non-functional proteins, potentially resulting in disease.
mRNA translation is a fundamental process in molecular biology that enables cells to convert the genetic information encoded in DNA into the functional proteins that drive all aspects of life. It is a complex and tightly regulated process, ensuring that the genetic blueprint is accurately translated into the proteins that shape our biological destiny.
mRNA Degradation: Managing the Cellular Message
Every cell in our bodies is a bustling hub of activity, with countless processes occurring simultaneously. Among these crucial processes is the degradation of mRNA, which plays a vital role in regulating gene expression and maintaining cellular homeostasis.
The Importance of mRNA Degradation
mRNA, or messenger RNA, carries the genetic instructions from DNA to the ribosomes, where proteins are synthesized. Once an mRNA molecule has served its purpose, it needs to be broken down and disposed of to make way for new mRNA molecules and prevent unnecessary protein production.
The Role of RNAse Enzymes
The degradation of mRNA is primarily carried out by a group of enzymes known as RNAse enzymes. These enzymes are molecular scissors that cleave the mRNA molecule into smaller fragments. There are different types of RNAse enzymes, each with its specific target and role in mRNA turnover.
Controlling mRNA Abundance
The degradation of mRNA is essential for controlling the abundance of specific proteins in the cell. By regulating the lifespan of mRNA molecules, cells can fine-tune the levels of proteins they produce. This allows cells to respond to changing conditions, such as environmental cues or developmental signals.
For example, if a cell needs more of a particular protein, it can increase the stability of the mRNA encoding that protein, thereby increasing protein production. Conversely, if a protein is no longer needed, the cell can degrade its mRNA to reduce protein synthesis.
Additional Considerations
In addition to the general role of mRNA degradation in gene regulation, there are specific instances where mRNA degradation is particularly important. For example, during development, the selective degradation of certain mRNAs can lead to the formation of different cell types and tissues.
mRNA degradation is an essential process that helps maintain cellular balance and allows cells to respond to their environment. By controlling the turnover and abundance of mRNA molecules, RNAse enzymes play a crucial role in regulating gene expression and shaping cellular function. Understanding the mechanisms of mRNA degradation is fundamental to unraveling the complexities of cellular life and developing new therapeutic strategies for a wide range of diseases.