Comprehending Genotypes: Unlocking The Secrets Of Genetic Inheritance And Expression
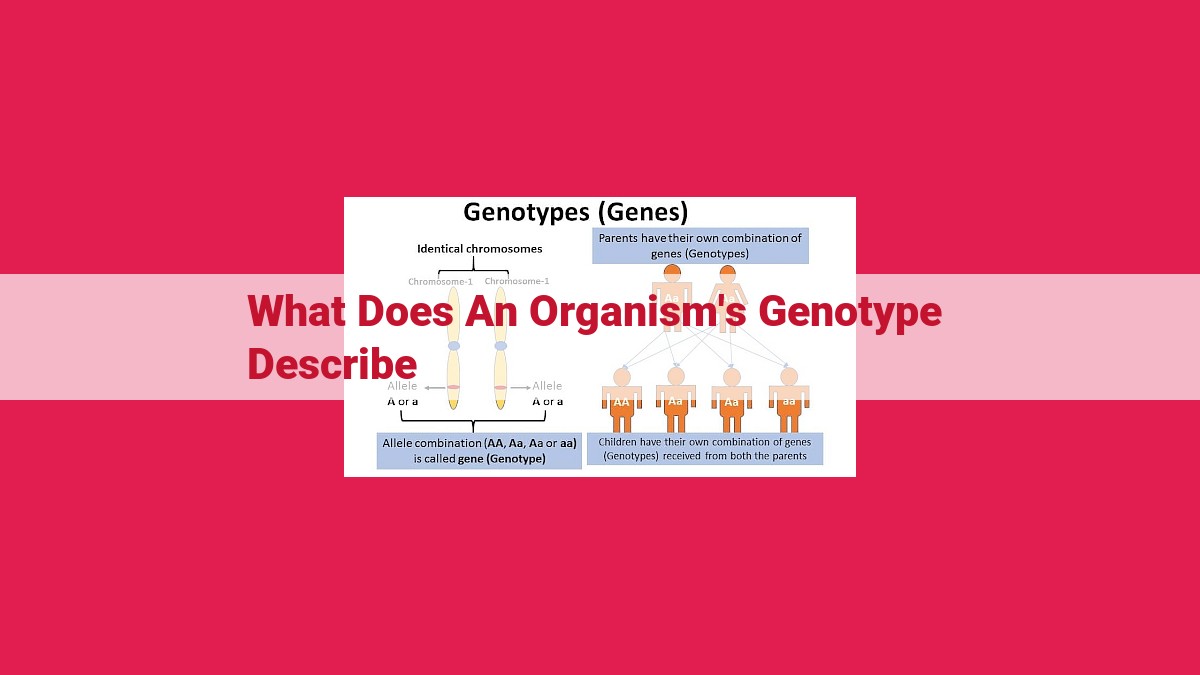
An organism’s genotype encompasses the complete set of its genetic information, comprising particular combinations of alleles—alternative forms of genes. This genetic makeup ultimately dictates an organism’s observable traits and characteristics. The genotype influences the organism’s phenotype (physical and biochemical expressions), with the interplay of dominant and recessive alleles determining the outcome. Understanding genotypes allows us to comprehend how genetic information is passed down through generations, aids in predicting the likelihood of inherited traits, and facilitates the identification and treatment of genetic disorders.
Understanding the Essence of an Organism’s Genotype
In the realm of biology, the genetic makeup of an organism holds immense significance. This genetic blueprint, known as the genotype, serves as the foundation for an organism’s unique identity, influencing traits and characteristics. It is a fundamental aspect of our existence, determining everything from our physical appearance to our predisposition to certain diseases.
Unveiling the Genotype’s Importance
The genotype serves as the blueprint for an organism’s traits, contributing to its distinctive features. For instance, the genotype determines the color of your eyes, the texture of your hair, and even aspects of your behavior. By understanding the genotype, we gain insights into the underlying genetic mechanisms that shape our individuality.
Key Components of a Genotype
- Alleles: Different forms of a gene
- Homozygous: Having two copies of the same allele
- Heterozygous: Having two different alleles
Key Components of an Organism’s Genotype
Unveiling the intricate tapestry of life, an organism’s genotype holds the blueprint for its unique traits and characteristics. Composed of a mosaic of alleles, these genetic fragments are the cornerstone of our genetic inheritance. Each gene, responsible for a specific aspect of our being, can manifest in various forms known as alleles.
Imagine a gene akin to a set of instructions for building a protein – the workhorse of our cells. Each allele, like a variation of these instructions, can guide the construction of slightly different versions of that protein. For instance, one allele may encode a brown-eyed protein, while another allele may blueprint blue eyes.
Just as genes come in pairs, so do alleles. An organism can be homozygous for a particular gene, possessing two identical alleles for that trait. This genetic uniformity leads to straightforward inheritance patterns – brown eyes from two brown-eyed alleles, blue eyes from two blue-eyed alleles.
However, when an organism inherits a different allele from each parent, it becomes heterozygous for that gene. This genetic diversity can result in more complex outcomes. For instance, an individual with one brown-eyed allele and one blue-eyed allele may express a mixture of both colors, resulting in hazel eyes.
Understanding Allele Interactions: A Tale of Dominance and Recession
In the realm of genetics, the interplay between different forms of a gene, known as alleles, plays a pivotal role in shaping an organism’s traits and characteristics. This interaction is characterized by two key concepts: dominance and recession.
Dominance: The King of the Alleles
Dominant alleles are powerful players in the genetic world. Their influence is so strong that they are expressed in the phenotype (observable characteristics) even when paired with a less assertive recessive allele. Think of a dominant allele as a charismatic leader, commanding the spotlight and dictating the observable traits of the organism.
Recession: The Silent Partner
Recessive alleles, on the other hand, are like shy introverts. They remain hidden and unexpressed when paired with a dominant allele. But when two recessive alleles come together, their true colors shine through. Recessive alleles whisper their hidden traits, revealing themselves in the organism’s phenotype.
A Genetic Dance of Expression
The relationship between dominant and recessive alleles is like a delicate dance. Dominant alleles take the lead, determining the phenotype. Recessive alleles wait patiently in the wings, only making an appearance when the stage is clear. This dance of dominance and recession creates a myriad of possible genetic combinations and phenotypic variations within populations.
Implications for Traits and Disorders
Understanding allele interactions is crucial for comprehending traits and genetic disorders. For example, brown eye color is dominant over blue eye color. If an individual has one allele for brown eyes and one for blue eyes, they will still have brown eyes because the brown allele dominates the phenotype.
Conversely, certain genetic disorders are only expressed when an individual inherits two copies of a recessive allele. Cystic fibrosis, a lung disease, is caused by a mutation in the CFTR gene. For an individual to develop cystic fibrosis, they must inherit two copies of the recessive allele responsible for the disorder.
Genotype and Phenotype: The Genetic Blueprint for Our Traits
Our genotype, the genetic blueprint we inherit from our parents, holds the key to our phenotype, the observable characteristics that make us unique. Like an intricate mosaic, our genotype influences everything from the color of our eyes to the structure of our bodies.
Imagine a jigsaw puzzle where each piece represents an allele, a variation of a gene. Our genotype is the complete set of puzzle pieces we receive, half from our mother and half from our father. The interaction of these alleles determines our phenotype, much like the way the puzzle pieces fit together to create a vivid image.
For example, let’s take eye color. Two alleles govern this trait: one for brown eyes and one for blue eyes. If an individual has two copies of the brown eye allele (homozygous for brown eyes), they will have brown eyes. If they receive one copy of each allele (heterozygous), their eyes will be blue. This demonstrates the remarkable influence of our genotype on our physical appearance.
Understanding the relationship between genotype and phenotype is crucial in medicine, forensics, and even selective breeding. By analyzing an individual’s genotype, scientists can gain insights into their health risks, identify potential genetic disorders, and trace genetic lineages. In agriculture, gene mapping techniques help breeders select plants with desirable traits, improving crop yields and resilience.
As advancements in genetic analysis continue to unfold, our ability to comprehend and manipulate genotypes becomes increasingly powerful. Technologies like CRISPR and genetic sequencing empower scientists to modify genes precisely, potentially opening up new avenues for treating genetic diseases and enhancing human health. However, these advancements also raise important ethical and societal considerations that must be carefully navigated.
By unraveling the intricate interplay between genotype and phenotype, we can gain a deeper understanding of ourselves and our place in the world. It is a testament to the extraordinary power of our genetic heritage, shaping who we are and paving the way for future generations to come.
Understanding Genotypes: A Guide to Predicting Offspring Traits Using Punnett Squares
In the realm of genetics, understanding an organism’s genotype, or genetic makeup, is crucial to deciphering its observable traits and characteristics. One powerful tool used to predict the possible genotypes and phenotypes of offspring is the Punnett square.
A Punnett square is a simple grid that helps visualize the possible combinations of alleles, inherited from each parent, that can result in the genotype of an individual. Alleles are different forms of a gene, which determine specific traits, such as eye color or height.
Homozygous individuals have two copies of the same allele, while heterozygous individuals possess two different alleles. Punnett squares are particularly useful for predicting the genotypes of heterozygous parents.
To construct a Punnett square, list the alleles inherited from each parent along the top and side of the grid. Each square within the grid represents the possible combinations of alleles that an offspring could inherit. The genotypes are represented on the top and left of the square, while the phenotypes, or observable traits, are typically indicated on the bottom and right.
For example, in a scenario where both parents are heterozygous for eye color, with one dominant allele (B) for brown eyes and one recessive allele (b) for blue eyes, the Punnett square would look as follows:
B | b |
---|---|
B | BB | Bb |
b | Bb | bb |
In this case, there are 25% chance that the offspring will be homozygous dominant (BB), with brown eyes. There is also a 25% chance of them being homozygous recessive (bb), with blue eyes. The remaining 50% of the offspring are expected to be heterozygous (Bb), inheriting one allele for brown eyes and one for blue eyes, resulting in brown eyes due to dominance.
By understanding the principles of Punnett squares, we can make probability-based calculations to predict the possible genotypes and phenotypes of offspring. This valuable tool has applications in genetic counseling, selective breeding, and even medicine.
Genetic Disorders: The Role of Genotypes in Diagnosis and Treatment
In the realm of genetics, understanding an organism’s genotype is crucial for unraveling the mysteries behind its traits and characteristics. Genotypes, which comprise the genetic makeup of individuals, play a pivotal role in determining not just our appearance but also our susceptibility to various health conditions.
Genetic disorders, a consequence of specific mutations or combinations of alleles, arise when the harmonious balance of our genetic code is disrupted. These mutations, like rogue messengers, can disrupt the functioning of essential genes, leading to a wide spectrum of health issues.
Recognizing the significance of genotypes in genetic disorders has revolutionized diagnosis and treatment strategies. Advanced genetic testing techniques, such as CRISPR and genetic sequencing, enable healthcare professionals to identify the precise genetic abnormalities responsible for these conditions. This knowledge empowers them to tailor personalized treatment plans, maximizing effectiveness and minimizing side effects.
For instance, in the case of cystic fibrosis, a life-threatening lung disease, identifying the specific genotype responsible for the disorder allows for targeted therapies that can improve lung function and extend life expectancy. Similarly, understanding the genetic underpinnings of sickle cell anemia has led to the development of gene therapies that aim to correct the underlying genetic defect.
The correlation between genotypes and genetic disorders highlights the immense potential of genetic research. By unraveling the intricacies of our genetic makeup, we unlock new avenues for diagnosis, develop innovative treatments, and ultimately pave the way for a healthier future.
Gene Mapping and Genotype Identification: Unraveling the Genetic Blueprint
In the realm of genetics, understanding an organism’s genotype is crucial to deciphering its physical and behavioral characteristics. Gene mapping and genotype identification provide invaluable tools for scientists and researchers to explore the intricate web of genes that contribute to our existence.
Gene Mapping: Pinpointing the Genetic Address
Like a roadmap, gene mapping guides scientists to the precise locations of genes on chromosomes. Using advanced techniques such as fluorescent tags and DNA sequencing, researchers can identify the specific sections of DNA responsible for particular traits and disorders. This detailed knowledge enables a deeper understanding of how genes exert their influence on our lives.
Genotype Identification: Connecting Genes to Traits
Once genes are mapped, genotype identification helps to determine the combination of alleles – alternative forms of genes – that an individual possesses. These genetic variations can have a profound impact on our physical features, susceptibility to diseases, and even our behavioral tendencies. By analyzing specific genetic markers, researchers can identify individuals at risk for certain conditions, guide treatment strategies, and predict the potential outcomes of genetic disorders.
Applications of Gene Mapping and Genotype Identification
Medicine: Gene mapping and genotype identification have revolutionized the field of medicine. By understanding the genetic basis of diseases, researchers can develop personalized treatments, predict disease risk, and screen for potential health issues. For example, identifying the BRCA1 and BRCA2 genes has significantly improved early detection and preventive measures for breast and ovarian cancer.
Forensics: In the realm of criminal investigations, gene mapping and genotype identification play a pivotal role. DNA profiling allows forensic scientists to identify individuals from blood, hair, or other biological samples, assisting in solving crimes and exonerating the innocent.
Selective Breeding: In the agricultural industry, gene mapping and genotype identification help farmers select livestock and crops with desirable traits. By identifying genetic markers linked to specific characteristics, such as increased milk production or disease resistance, breeders can improve the overall quality and productivity of their livestock and crops.
Advancements and Ethical Considerations
The field of gene mapping and genotype identification continues to advance at a rapid pace. Techniques like CRISPR and genetic sequencing are enabling scientists to manipulate and repair genes, raising both exciting possibilities and ethical concerns. As we delve deeper into the genetic realm, it is imperative to engage in thoughtful discussions and regulations to ensure the responsible use of these powerful technologies.
Advancements in Genotype Analysis: Unlocking the Secrets of Our Genetic Code
In the realm of genetics, advancements in genotype analysis have propelled us forward, empowering us with unprecedented knowledge and control over our genetic heritage. These cutting-edge technologies, such as CRISPR and genetic sequencing, are revolutionizing our understanding of genotypes and their profound impact on our lives.
CRISPR: A Gene-Editing Precision Tool
CRISPR-Cas9, an innovative gene-editing technology, allows scientists to precisely modify specific sections of DNA. This tool has unleashed a new era of genetic engineering, enabling researchers to correct gene defects, study gene function, and potentially treat a wide range of genetic disorders.
Genetic Sequencing: Unraveling the Genetic Blueprint
Genetic sequencing technologies, such as next-generation sequencing (NGS), have made it possible to rapidly and accurately determine the sequence of nucleotides that make up our DNA. By analyzing these sequences, scientists can identify genetic variations associated with health conditions, determine genetic risk factors, and develop personalized treatments.
Ethical and Societal Considerations
While these advancements offer immense potential for improving human health and understanding, they also evoke ethical and societal concerns. The ability to manipulate genotypes raises questions about the potential for genetic enhancement, equitable access to genetic information, and the potential misuse of genetic data.
Gene Mapping and Selective Breeding
Advancements in genotype analysis have also enabled the development of gene mapping techniques. By identifying the location of genes responsible for specific traits, scientists can gain insights into the genetic basis of complex diseases, develop diagnostic tests, and even use selective breeding to improve agricultural yields.
As we continue to unravel the mysteries of our genetic code, these groundbreaking technologies hold the promise of transforming medicine, agriculture, and our fundamental understanding of human biology. However, it is crucial to engage in responsible and ethical discussions to ensure that the advancements in genotype analysis are used for the betterment of humankind, safeguarding the integrity of our DNA and the equity of access to genetic information.