Chromium Oxidation State: Key Factors And Implications For Chemical Behavior
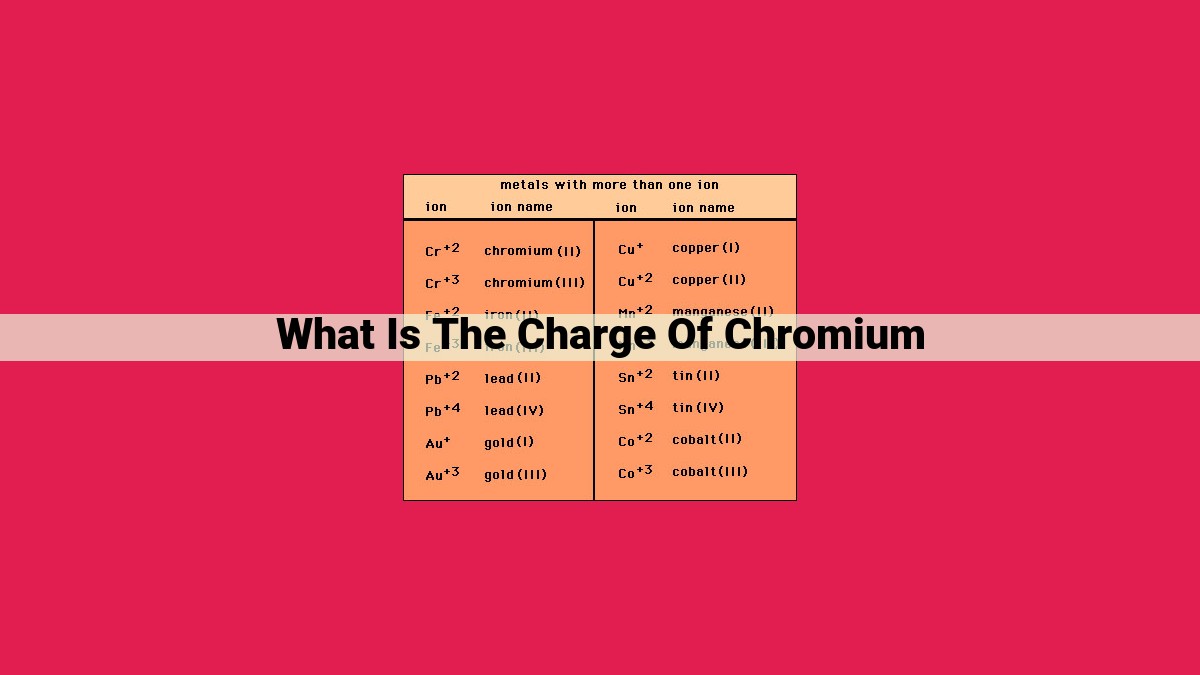
Chromium’s oxidation state, dictated by its electron configuration and valence electrons, is influenced by factors like ionization energy, electronegativity, and periodic trends. Its oxidation state determines its positive or negative charge, reflecting the number of valence electrons lost or gained. Understanding these factors is crucial for predicting chromium’s chemical behavior, as they govern its bonding potential, reactivity, and interactions with other elements.
Understanding the Oxidation State of Chromium
In the realm of chemistry, understanding the oxidation state of an element is crucial to unraveling its chemical behavior. It delves into the fundamental nature of the element, revealing the charge it can exhibit in chemical compounds. Oxidation state refers to the hypothetical charge an atom would have if all its electrons were transferred to the most electronegative atoms in the compound.
Chromium, a transition metal with a silvery-white appearance, exhibits a range of oxidation states, showcasing its versatility in chemical bonding. The most common oxidation states of chromium are +2, +3, and +6, although it can also exhibit other oxidation states under specific conditions.
The oxidation state of chromium is influenced by several factors, including its electron configuration, ionization energy, and electronegativity. These properties work in conjunction to determine the tendency of chromium to lose or gain electrons, thereby influencing its charge.
Chromium’s Electron Configuration and Oxidation State
The electron configuration of chromium is [Ar] 3d5 4s1, indicating that it has six valence electrons. These valence electrons are readily lost or shared in chemical reactions, giving rise to different oxidation states. For instance, when chromium loses two electrons, it attains an oxidation state of +2. This oxidation state is commonly observed in compounds such as chromium(II) chloride (CrCl2).
Ionization Energy and Electronegativity
Ionization energy measures the energy required to remove an electron from an atom. Chromium’s relatively high ionization energy indicates that it has a strong hold on its electrons, making it less likely to lose them. This property contributes to chromium’s stability in the +3 oxidation state, as seen in compounds like chromium(III) oxide (Cr2O3).
Electronegativity, on the other hand, quantifies an atom’s ability to attract electrons towards itself. Chromium’s moderate electronegativity suggests that it has a balanced tendency to attract and release electrons. This balance allows chromium to exhibit multiple oxidation states, depending on the electronegativity of the elements it bonds with.
Valence Electrons and Oxidation State
The number of valence electrons that chromium loses or gains directly corresponds to its oxidation state. When chromium loses two electrons, it attains an oxidation state of +2, indicating that it has lost two positive charges. Conversely, if chromium gains three electrons, it reaches an oxidation state of -3, suggesting that it has acquired three negative charges.
Periodic Trends
The oxidation state of chromium also follows periodic trends. Elements in the same group tend to exhibit similar oxidation states due to their comparable electron configurations. For instance, chromium belongs to Group 6, which includes elements such as molybdenum and tungsten. These elements commonly exhibit oxidation states of +2, +3, and +6, reflecting their shared chemical properties.
In conclusion, understanding the oxidation state of chromium provides valuable insights into its chemical behavior. By considering factors such as electron configuration, ionization energy, and electronegativity, we can predict the charge that chromium will exhibit in various compounds. This knowledge is essential for unraveling the complex interactions that occur in chemical reactions, enabling us to harness the unique properties of chromium in numerous technological applications.
Electron Configuration and Valence Electrons: Unraveling Chromium’s Chemical Identity
Chromium’s Electron Configuration: A Blueprint of Chemical Potential
Every element’s dance with other atoms is dictated by its electron configuration – the arrangement of its electrons in different energy levels. For chromium, this blueprint reads as follows: [Ar] 3d⁵ 4s¹. This configuration tells us a captivating tale of its chemical personality.
The 3d⁵ signifies the presence of five electrons in the d orbitals, which are responsible for chromium’s unique magnetic properties. These electrons form an intriguing dance within the atom, shaping its bonding behavior and influencing its oxidation states.
Moreover, the 4s¹ indicates a lone electron in the outermost energy level, eagerly seeking to participate in chemical transformations. This electron plays a crucial role in determining chromium’s valence – the number of bonds it can form – and its ability to donate or accept electrons, giving rise to its diverse oxidation states.
Valence Electrons: The Key to Chromium’s Bonding Adventures
These valence electrons, like mischievous imps, hold the key to chromium’s bonding potential. Their number and energy determine the element’s eagerness to form chemical bonds. Chromium’s single valence electron grants it the ability to forge bonds with other atoms, seeking stability and a harmonious balance of electrons.
The significance of valence electrons extends beyond bond formation. They also play a pivotal role in determining chromium’s oxidation state – the perceived charge on the element when it participates in chemical reactions. By losing or gaining electrons, chromium’s valence electrons dictate its oxidation state, allowing it to dance with other elements in a variety of ways.
Ionization Energy: Unlocking Chromium’s Inner Strength
Ionization energy is the energy required to remove an electron from an atom, a measure of how tightly electrons are held by the atom. Chromium‘s ionization energy is relatively high, indicating that its electrons are strongly bound. This high ionization energy suggests that chromium is less likely to lose electrons and form positive ions.
Electronegativity: Chromium’s Affinity for Electrons
Electronegativity measures an atom’s ability to attract valence electrons. Chromium has a moderate electronegativity, meaning it can both donate and accept electrons, depending on its chemical environment. This balanced electronegativity makes chromium versatile in forming chemical bonds.
The Dance of Ionization Energy and Electronegativity
The interplay between ionization energy and electronegativity shapes chromium’s oxidation state. Oxidation state refers to the charge assigned to an element in a compound. Chromium can exhibit various oxidation states, but its most common ones are +2, +3, and +6.
-
+2 oxidation state: Chromium loses two electrons, resulting in two positive charges. This state occurs when chromium’s ionization energy is overcome, allowing it to donate two electrons to achieve stability.
-
+3 oxidation state: Chromium loses three electrons, creating three positive charges. This state is achieved when chromium’s ionization energy is partially overcome, allowing it to donate three electrons while retaining some electrons for bonding.
-
+6 oxidation state: Chromium loses six electrons, resulting in six positive charges. This state occurs only in compounds with highly electronegative elements, such as oxygen, which strongly attract chromium’s valence electrons.
The balance between ionization energy and electronegativity determines which oxidation state is most favorable for chromium in a particular chemical environment.
Valence Electrons and Oxidation State: The Key to Chromium’s Chemical Identity
In the realm of chemistry, understanding the oxidation state of an element is crucial for predicting its chemical behavior. It reveals the charge of the element, providing insights into its bonding potential and reactivity. In this context, the valence electrons of chromium play a pivotal role in determining its oxidation state.
Chromium’s Electron Configuration and Valence Electrons:
Chromium, with an atomic number 24, possesses 24 electrons and is located in group 6 of the periodic table. Its electron configuration is [Ar]3d5 4s1
. The valence electrons are the outermost electrons, which in chromium’s case are the one electron in the 4s
orbital and the five electrons in the 3d
orbital.
Oxidation State and Valence Electrons:
The oxidation state of an element represents the number of electrons it gains or loses to achieve a stable electron configuration. For chromium, a positive oxidation state indicates that it has lost electrons, while a negative oxidation state indicates it has gained electrons.
The number of valence electrons that chromium loses or gains corresponds to its oxidation state. For example, if chromium loses three electrons (oxidation state +3), it has three fewer valence electrons, resulting in the electron configuration [Ar]3d2
. Conversely, if chromium gains three electrons (oxidation state -3), it has three more valence electrons, leading to the electron configuration [Ar]3d8
.
Interconnection with Ionization Energy and Electronegativity:
The ionization energy of an element is the energy required to remove an electron. The electronegativity measures the ability of an element to attract electrons. These properties influence the oxidation state of chromium.
A high ionization energy means that it is difficult for chromium to lose electrons, leading to a more positive oxidation state. Conversely, a low electronegativity means that chromium has a lower tendency to attract electrons, again resulting in a more positive oxidation state.
Valence electrons are fundamental to the oxidation state of chromium. The number of valence electrons lost or gained corresponds to the oxidation state, and this concept is closely interconnected with ionization energy and electronegativity. By understanding these relationships, we gain a deeper insight into the chemical identity and behavior of chromium.
Periodic Trends: A Compass for Chromium’s Oxidation State
Every element in the periodic table has a unique fingerprint that governs its chemical behavior. For chromium, this fingerprint is shaped by its position, atomic number, and mass, which together orchestrate its electron configuration and ultimately its oxidation state.
Periodic trends are the predictable patterns that emerge as we traverse the periodic table. These trends allow us to forecast an element’s properties based on its location. Chromium, residing in Group 6 of the periodic table, exhibits intriguing trends that influence its oxidation state.
With an atomic number of 24, chromium ranks among the higher-numbered elements. This means its electrons are farther from the nucleus, making them more easily lost or gained during chemical reactions. This behavior profoundly impacts the oxidation state of chromium.
As we move from left to right within a period, the number of protons in the nucleus increases, drawing the electrons closer. This results in higher ionization energies, meaning more energy is required to remove an electron. Conversely, as we move down a group, the number of electron shells increases, distancing the electrons from the nucleus and lowering ionization energies.
Chromium’s position within the periodic table reflects these trends. Its higher atomic number necessitates greater energy to remove electrons, while its location in Group 6 indicates a relatively low ionization energy due to its position within the period.
These periodic trends collectively shape the electron configuration of chromium. Its valence electrons, the outermost electrons involved in bonding, determine the element’s bonding potential and oxidation state. Chromium’s six valence electrons allow it to assume various oxidation states, ranging from -2 to +6, with the most common being +2, +3, and +6.
Understanding periodic trends is crucial for predicting the chemical behavior of chromium. By unraveling the tapestry of its position, atomic number, and mass within the periodic table, we gain insights into the factors that dictate its oxidation state and chemical interactions. This knowledge forms the cornerstone for comprehending the multifaceted nature of chromium and its remarkable versatility in various chemical contexts.