Understanding Chloroplasts: Essential Energy Converters In Plant Cells
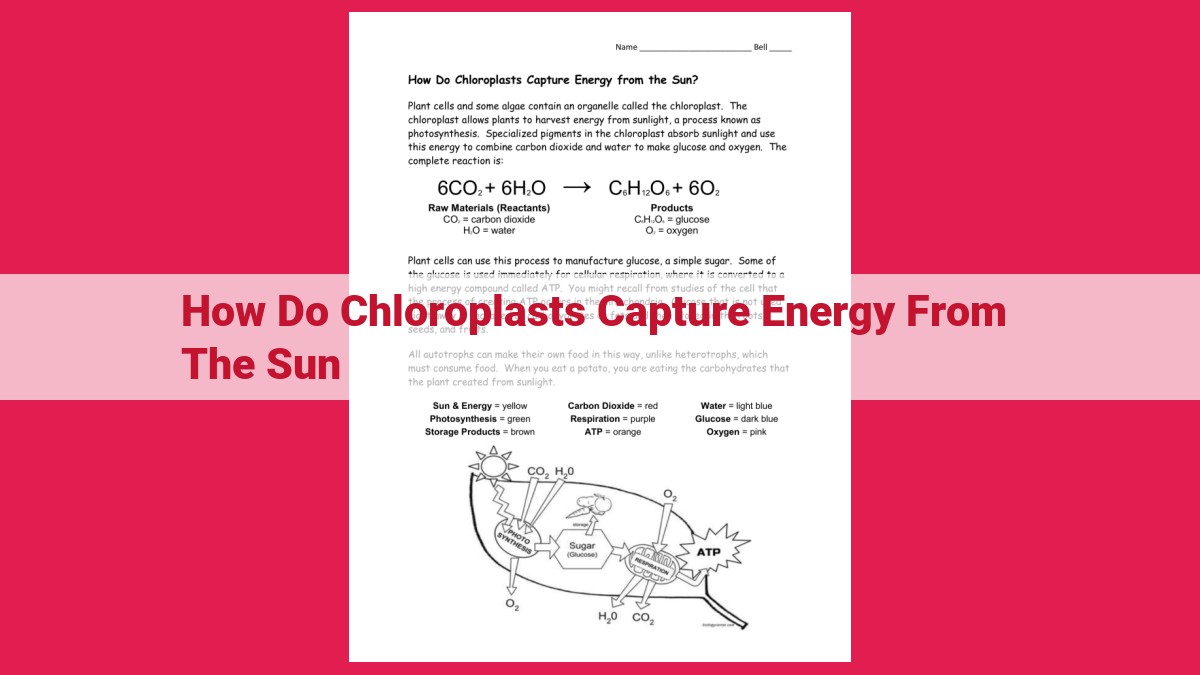
Chloroplasts, the energy-capturing organelles in plant cells, harness sunlight through pigments like chlorophyll. These pigments absorb specific wavelengths of light, transferring energy to electrons in a series of light-dependent reactions. These reactions split water and generate ATP and NADPH, energy carriers used in light-independent reactions (Calvin cycle) to fix carbon dioxide into glucose.
Photosynthesis: The Energy-Capturing Marvel
In the enchanting realm of the natural world, where verdant leaves dance in unison, a remarkable process known as photosynthesis unfolds, transforming sunlight into life-sustaining energy. It’s a complex dance of nature, where plants harness the sun’s rays to create their own sustenance, fueling the very fabric of our planet.
At the heart of this photosynthetic alchemy lies the chloroplast, a miniature marvel within the plant cells. These tiny organelles are veritable powerhouses, adorned with pigments that act as solar panels, absorbing the sun’s energy. These pigments, like the vibrant chlorophyll, are essential players in this energetic drama.
Sunlight, upon striking the chlorophyll, triggers a light-dependent reaction. It’s a symphony of electron transfer, water splitting, and the miraculous production of ATP (the energy currency of the cell) and NADPH (the electron carrier).
The next act in this photosynthetic play is the light-independent reaction (also known as the Calvin cycle). Armed with ATP and NADPH, the plant proceeds to capture carbon dioxide from the atmosphere, converting it into the sweet nectar of life: glucose.
Glucose, the fundamental building block of plant life, serves as the foundation for the intricate web of life on Earth. Animals, including us humans, ultimately derive our sustenance from this photosynthetic marvel, showcasing the interconnectedness of nature’s tapestry.
So, there you have it, the captivating tale of photosynthesis, a testament to nature’s ingenuity and the boundless energy that sustains our planet. It’s a process that not only nourishes plants but also weaves the very fabric of life, from the tiniest insects to the grandest whales.
Chloroplasts: The Photosynthesis Powerhouse
Imagine these tiny organelles within plant cells as the engines that fuel life on Earth. Chloroplasts, the green powerhouses of photosynthesis, are the sites where sunlight’s energy is transformed into the chemical energy that sustains us all.
Chloroplast Structure: A Microscopic Laboratory
These organelles have a complex internal structure that allows them to efficiently capture and process light energy. They are surrounded by a double membrane, with an intermembrane space between them. Inside the chloroplast, thylakoids are stacked together to form grana, which are arranged like pancakes in a stack. The space outside the thylakoids is called stroma.
Pigments: Nature’s Light Absorbers
Within the thylakoid membranes reside pigments, molecules that absorb specific wavelengths of light. Chlorophyll, the primary pigment, absorbs blue and red light, while carotenoids and phycobilins absorb other wavelengths. This absorption of light provides the energy that drives the chemical reactions of photosynthesis.
Thylakoids and Stroma: The Reaction Zone
Thylakoids are the sites of the light-dependent reactions, where light energy is converted into ATP and NADPH. The stroma is where the light-independent reactions (also known as the Calvin cycle) take place. Here, ATP and NADPH are used to assimilate carbon dioxide into glucose, the energy-rich molecule that fuels plant growth and provides food for all living organisms.
Chloroplasts: The Foundation of Life
Chloroplasts are essential for life on our planet. They not only provide the food that sustains us but also release oxygen into the atmosphere. Without chloroplasts and photosynthesis, life as we know it would simply not exist.
Pigments: The Colorful Symphony of Sunlight Absorption
In the realm of photosynthesis, pigments play a pivotal role in capturing the radiant energy of the sun. They are the pigments that give plants their verdant hues, and their ability to harness the power of sunlight is essential for the survival of all life on Earth.
Types of Pigments
The photosynthetic world boasts a kaleidoscope of pigments, each with its unique spectral affinity. Among them, chlorophyll reigns supreme, absorbing the vast majority of sunlight. Carotenoids, with their vibrant hues of yellow, orange, and red, play a supporting role, absorbing the remaining wavelengths that chlorophyll misses. Phycobilins, found in cyanobacteria and certain algae, also contribute to the photosynthetic symphony, capturing blue and red light.
Absorption and Excitation
These pigments act as tiny light-gathering antennas, capturing photons of sunlight and converting their energy into excited electrons. Chlorophyll, with its sophisticated structure, efficiently traps red and blue light, while carotenoids and phycobilins target specific wavelengths.
Passing the Torch
Once excited, these electrons embark on a remarkable journey, passing their energy from pigment to pigment. This energy transfer creates an electron transport chain, which ultimately drives the chemical reactions of photosynthesis.
In conclusion, pigments are the vibrant gatekeepers of photosynthesis, absorbing the solar spectrum and initiating the vital energy-capturing process. Their presence not only paints the natural world in a myriad of colors but also sustains the delicate balance of life on our planet.
Chlorophyll: The Primary Light Absorber
In the vibrant tapestry of life, chlorophyll stands out as the maestro of photosynthesis, the enchanting process that transforms sunlight into life-sustaining energy. This remarkable pigment, dwelling within the heart of chloroplasts, is the orchestra conductor that orchestrates the transformation of light into the vital energy that fuels the biosphere.
Chlorophyll’s structure is an intricate symphony of nature’s artistry. At its core lies a molecule called porphyrin, a flat, ring-shaped structure adorned with a magnesium ion. Attached to this ring is a long, hydrocarbon chain known as a phytol tail, which anchors chlorophyll within the chloroplast’s membranes.
The magic of chlorophyll lies in its ability to absorb sunlight. When a photon of light strikes a chlorophyll molecule, it excites an electron within the molecule. This energized electron embarks on a perilous journey, passing through a series of molecules within the thylakoid membranes of the chloroplast. As the electron races along, its energy is harnessed to pump protons across the membrane, creating a proton gradient.
The proton gradient is the driving force behind the synthesis of ATP, the primary energy currency of all living cells. As protons flow back across the membrane through a specialized channel, their energy is captured and used to attach phosphate molecules to ADP, forming ATP.
Simultaneously, the excited electron is transferred to NADP+, a molecule that serves as an electron carrier. This NADPH molecule will play a crucial role in the light-independent reactions of photosynthesis, where the energy stored in ATP and NADPH is used to convert carbon dioxide into glucose.
Without chlorophyll, the photosynthetic symphony would come to an abrupt halt. It is the prima donna of light absorption, the maestro that orchestrates the conversion of sunlight into life-sustaining energy.
Light-Dependent Reactions: Generating Energy Carriers
In the realm of photosynthesis, where plants harness the sun’s energy, light-dependent reactions play a pivotal role in generating the energy carriers that fuel life on Earth. These reactions, occurring within the chloroplasts’ thylakoids, are a symphony of electron transfer, water splitting, and ATP synthesis.
Electron Transfer: The Energy Relay
The light-dependent reactions begin with the absorption of light energy by chlorophyll pigments. This energy excites electrons, which are then passed along a series of electron carriers. As electrons cascade through this chain, they release energy, which is used to pump protons across the thylakoid membrane. This creates a proton gradient, which serves as the driving force for ATP synthesis.
Water Splitting: The Oxygen Source
Simultaneously, water molecules are split, releasing oxygen as a byproduct and generating electrons and protons. These electrons also enter the electron transfer chain, contributing to the proton gradient. The oxygen released by water splitting diffuses into the atmosphere, essential for life on the planet.
ATP Synthesis: The Energy Currency
The culmination of these processes is the synthesis of adenosine triphosphate (ATP), the cellular energy currency. As protons flow down the proton gradient through ATP synthase, an enzyme embedded in the thylakoid membrane, the energy released is used to convert ADP (adenosine diphosphate) into ATP. ATP, the primary energy carrier in cells, powers numerous cellular processes, including carbon fixation in the subsequent light-independent reactions.
Light-Independent Reactions: Harnessing Energy for Life
The Calvin Cycle: A Carbon-Fixing Marathon
After capturing sunlight’s energy through light-dependent reactions, plants embark on a crucial mission: fixing atmospheric carbon into organic molecules. This process, known as the Calvin cycle, unfolds in the stroma of chloroplasts, where carbon atoms are transformed into the building blocks of life.
Enlisting the Power of ATP and NADPH
The Calvin cycle relies on the energy carriers produced in light-dependent reactions. ATP (adenosine triphosphate) serves as an energy currency, providing the power for chemical reactions. NADPH (nicotinamide adenine dinucleotide phosphate), an electron carrier, supplies the electrons necessary for carbon fixation.
A Step-by-Step Odyssey
The Calvin cycle consists of three main stages. First, carbon dioxide (CO2) from the atmosphere is captured and attached to a molecule called ribulose 1,5-bisphosphate (RuBP). This process, known as carbon fixation, is catalyzed by the enzyme RuBisCO.
Next, the six-carbon molecule created in carbon fixation is split into two three-carbon molecules, which then receive electrons from NADPH and ATP. This series of reactions reduces the carbon atoms and prepares them for synthesis.
In the final stage, the three-carbon molecules are rearranged and combined to form glucose, a six-carbon sugar that serves as the primary energy source for plants and other organisms.
The Importance of Glucose
Glucose is the fuel that powers the growth, development, and function of plants. It provides the energy needed for cellular respiration and the building blocks for the synthesis of complex carbohydrates, proteins, and lipids. Without the Calvin cycle, plants would be unable to harness the sun’s energy and sustain life on Earth.
ATP and NADPH: The Energy Currency and Electron Carrier
In the intricate ballet of life, ATP and NADPH play starring roles as the energy currency and electron carrier of all living cells. These molecules power the intricate dance of biological processes, providing the energy and electrons necessary for countless cellular functions.
ATP (adenosine triphosphate) is the universal energy molecule of all living organisms. Its structure resembles a tiny battery, with a sugar backbone, an adenine base, and three phosphate groups. The energy stored in the bonds between these phosphates acts as a ready source of power for a vast array of cellular processes, including muscle contraction, cell division, and protein synthesis.
NADPH (nicotinamide adenine dinucleotide phosphate) is an electron carrier that plays a crucial role in photosynthesis and other metabolic pathways. It ferries electrons from one molecule to another, enabling chemical reactions to occur and energy to be generated. Its presence is indispensable for the reduction reactions involved in processes such as photosynthesis and the synthesis of lipids and nucleic acids.
Both ATP and NADPH are vital for cellular metabolism. ATP provides the energy required for cells to carry out their functions, while NADPH provides the electrons for chemical reactions. In photosynthesis, light energy is captured and used to generate ATP and NADPH, which are then employed to convert carbon dioxide into glucose, the building block of life.
The importance of ATP and NADPH extends far beyond cellular metabolism. They also play critical roles in DNA replication, RNA transcription, and protein translation. These fundamental processes are essential for growth, development, and the maintenance of life.
Without ATP and NADPH, the dance of life would cease. These remarkable molecules are the driving force behind the countless cellular processes that sustain life on Earth. Their importance cannot be overstated. By understanding their roles, we gain a deeper appreciation for the intricate workings of life and the incredible complexity that underlies even the most basic of organisms.