Understanding Chemical Behavior Through Electron Dot Structures: A Guide For Beginners
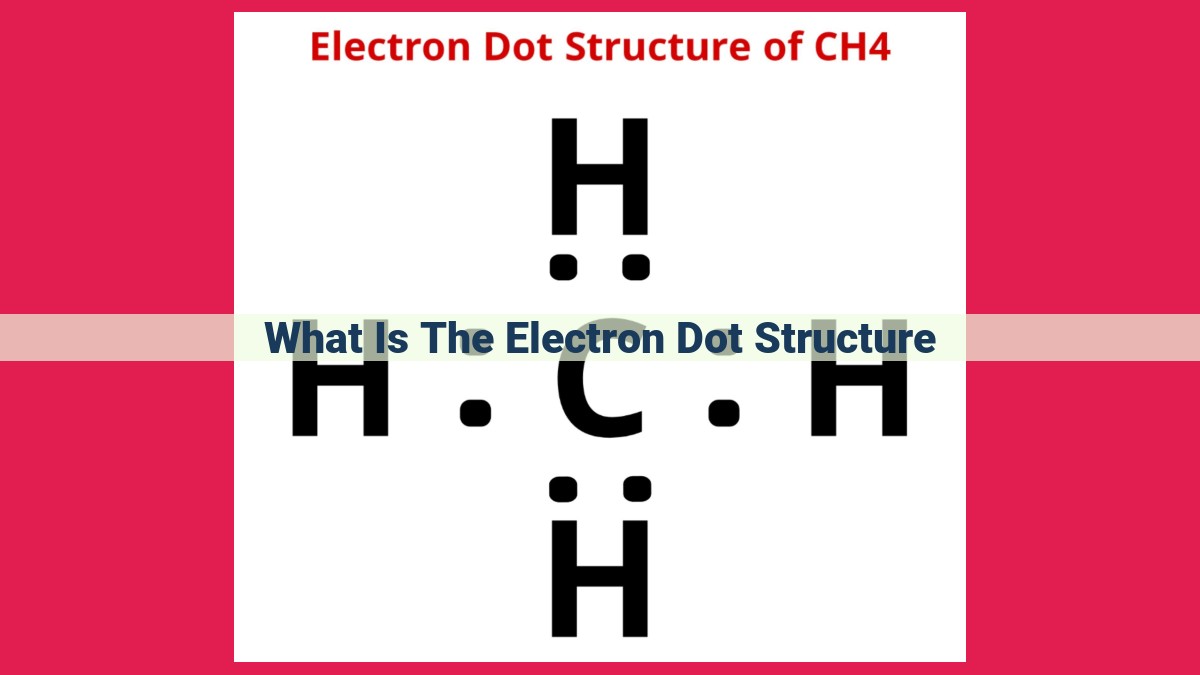
Electron dot structures, a representation of the valence electrons in an atom or molecule, are crucial for understanding their chemical behavior. Valence electrons, the outermost electrons, determine an atom’s bonding tendencies, while core electrons remain stable within the atom. By following the octet rule, atoms strive to have eight valence electrons around them, leading to the formation of chemical bonds. Electron dot structures depict these valence electrons as dots around the element symbol and can predict molecular geometry using VSEPR theory.
Electron Dot Structures: Unveiling the Language of Atoms
In the captivating world of chemistry, atoms communicate through a symphony of electrons. Their dance around atomic nuclei holds the secrets to the formation of molecules and the symphony of life. Electron dot structures, also known as Lewis dot structures, provide a visual representation of this atomic dialogue.
These enigmatic diagrams are like maps that guide us through the electron landscape of atoms. They reveal the number and arrangement of valence electrons, the mischievous electrons that roam the outermost energy level of an atom. By understanding the patterns and principles of electron dot structures, we can decipher the language of atoms and unravel the secrets of their chemical interactions.
Electron dot structures not only depict the electron distribution but also predict the stability and behavior of atoms. The octet rule, a guiding principle in this atomic dialect, dictates that atoms seek stability by acquiring eight valence electrons. This explains why so many molecules exist with a complete octet of valence electrons, such as the ubiquitous water molecule (H2O).
Valence Electrons: The Kingmakers of Chemistry
In the realm of chemistry, where atoms dance and form countless bonds, there exists a special group of electrons that hold the key to understanding their behavior: valence electrons. Picture them as the energetic and sociable members of the atomic family, eager to interact with their surroundings.
These valence electrons reside in the outermost energy level of an atom, like mischievous kids playing on the playground. They’re the ones that determine an atom’s chemical properties, influencing its ability to form bonds with other atoms. The number of valence electrons an atom possesses dictates its eagerness to participate in chemical reactions, making them the true gatekeepers of atomic interactions.
Valence Electrons and Chemical Bonding: A Delicate Dance
The quest for stability drives atoms to seek a complete outer energy level, which often means acquiring or sharing valence electrons. Valence electrons act as the bridge between atoms, allowing them to form chemical bonds and create the myriad molecules and compounds that shape our world. By understanding the number and arrangement of valence electrons, chemists can unravel the mysteries of chemical reactions and predict the properties of new molecules.
Examples of Valence Electron Influence
- Helium, with its two valence electrons, is a noble gas known for its aloofness. It’s content with its stable octet of electrons, making it reluctant to participate in chemical reactions.
- Sodium, on the other hand, is highly reactive due to its single valence electron. Eager to shed this extra electron, sodium readily forms bonds with other atoms, such as chlorine, to achieve stability.
- Oxygen, with its six valence electrons, needs two more to complete its octet. This makes oxygen a powerful oxidizing agent, readily accepting electrons from other atoms.
Valence electrons are the unsung heroes of chemistry, playing a crucial role in determining an atom’s chemical behavior and influencing the formation of countless molecules. Understanding the principles of valence electrons is a cornerstone of chemistry, unlocking the ability to predict reactions, create new materials, and explore the fascinating world of atomic interactions.
Core Electrons: The Stable Foundation of Atoms
Nestled within the protonic heart of every atom lies a crucial layer of stability, often overlooked yet indispensable: the core electrons. These silent guardians, unlike their adventurous valence counterparts, dwell deep within the atom’s core, far removed from the action of chemical bonding.
Their secluded existence ensures their unwavering stability. Core electrons dance in tightly bound orbits, held captive by the powerful gravitational pull of the atom’s positively charged nucleus. This nuclear embrace shields them from the whims of outer electrons, allowing them to maintain their constant energy levels.
Unlike valence electrons, which eagerly participate in chemical reactions, core electrons serve as unwavering anchors. They steadily orbit the nucleus, providing a stable foundation for the atom. Their presence is vital for maintaining the atom’s overall structure and identity.
In essence, core electrons act as the silent guardians of the atom. They provide the stability and framework upon which chemical reactions occur. Without their unwavering presence, atoms would lose their structural integrity and the dance of chemistry would cease to exist.
The Octet Rule: A Quest for Stability in Electron Dot Structures
In the realm of chemistry, atoms strive to achieve a harmonious dance of electrons, seeking the elusive octet rule. This rule governs the behavior of atoms, dictating their quest for stability.
The octet rule proclaims that an atom attains stability when its outermost electron shell is filled with eight electrons. This is a magical number, a chemical Holy Grail, responsible for shaping the structure and properties of numerous molecules.
Atoms with a complete octet in their outermost shell possess a sense of fulfillment, like a contented puzzle with all its pieces in place. They are less likely to react, as they have no desire to gain or lose electrons. They have found their equilibrium, their perfect balance.
In electron dot structures, which depict the arrangement of electrons around atoms, the octet rule is manifested in the octet of dots surrounding certain atoms. These dots represent the valence electrons, the electrons that actively participate in bonding.
The octet rule is not an absolute law, however. Some atoms, particularly those with smaller atomic number, can achieve stability with less than an octet in their outermost shell. They are content with a duet, a pair of electrons, or a sextet, a group of six.
The octet rule is a powerful guide, providing insight into the behavior of atoms and the formation of chemical bonds. It helps scientists understand the intricate tapestry of molecular structures that make up the world around us.
Lewis Symbols: Unveiling the Secrets of Electron Dot Structures
In the realm of chemistry, understanding the intricacies of atoms and molecules is essential. Electron dot structures, a fundamental tool in this exploration, provide a visual representation of valence electrons. These outermost electrons determine the chemical behavior of elements and shape the molecular landscapes we encounter.
To simplify the representation of electron dot structures, scientists have devised a shorthand notation known as Lewis symbols. These symbols consist of the element’s chemical symbol surrounded by dots, each dot representing a single valence electron. Lewis symbols offer a convenient way to predict and depict electron dot structures.
Consider the example of carbon. Its atomic number is 6, indicating that it has six electrons. Four of these are valence electrons, which are represented as four dots in its Lewis symbol:
:C:
Similarly, nitrogen has five valence electrons, represented as three dots in its Lewis symbol:
:N:
Lewis symbols provide a rapid and efficient method for visualizing the valence electrons of various elements. By arranging these symbols according to the principles of electron dot structures, scientists can gain insights into molecular bonding and chemical reactivity. The use of Lewis symbols continues to play a vital role in advancing our understanding of the world of atoms and molecules.
VSEPR Theory: Unveiling the Secrets of Molecular Geometry
In the realm of chemistry, understanding the three-dimensional shape of molecules holds great significance. This is where VSEPR theory, an acronym for Valence Shell Electron Pair Repulsion, enters the picture. It’s a powerful tool that helps us predict the geometry of molecules based on the arrangement of valence electrons.
VSEPR theory is predicated on the idea of electron-pair repulsion. The valence electrons in a molecule tend to repel each other, seeking the most stable configuration that minimizes this repulsion. This results in specific molecular geometries determined by the number of electron pairs around the central atom.
Linear Geometry:
- For a central atom with two electron pairs, the linear geometry is adopted. This occurs when the electron pairs are oriented 180° apart, maximizing their distance and minimizing repulsion. Examples include carbon dioxide (CO2) and hydrogen cyanide (HCN).
Trigonal Planar Geometry:
- When a central atom has three electron pairs, it adopts a trigonal planar geometry. The electron pairs arrange themselves in a plane, forming 120° angles to minimize repulsion. Examples of molecules with this geometry are boron trifluoride (BF3) and ozone (O3).
Tetrahedral Geometry:
- For four electron pairs around a central atom, the tetrahedral geometry is most stable. The electron pairs occupy the corners of a tetrahedron, forming 109.5° angles to minimize repulsion. Examples include methane (CH4) and ammonia (NH3).
Trigonal Bipyramidal Geometry:
- When five electron pairs surround a central atom, the trigonal bipyramidal geometry is adopted. The electron pairs arrange themselves in a trigonal pyramid with an additional pair occupying an axial position. An example of this is phosphorus pentachloride (PCl5).
Octahedral Geometry:
- For six electron pairs around a central atom, the octahedral geometry is most stable. The electron pairs occupy the corners of an octahedron, forming 90° angles to minimize repulsion. An example of an octahedral molecule is sulfur hexafluoride (SF6).
By understanding VSEPR theory, we can predict the molecular geometry of a vast array of compounds. This knowledge is essential for understanding chemical bonding, molecular interactions, and the physical properties of materials.