Cellular Respiration Vs. Fermentation: Understanding The Key Differences For Atp Production
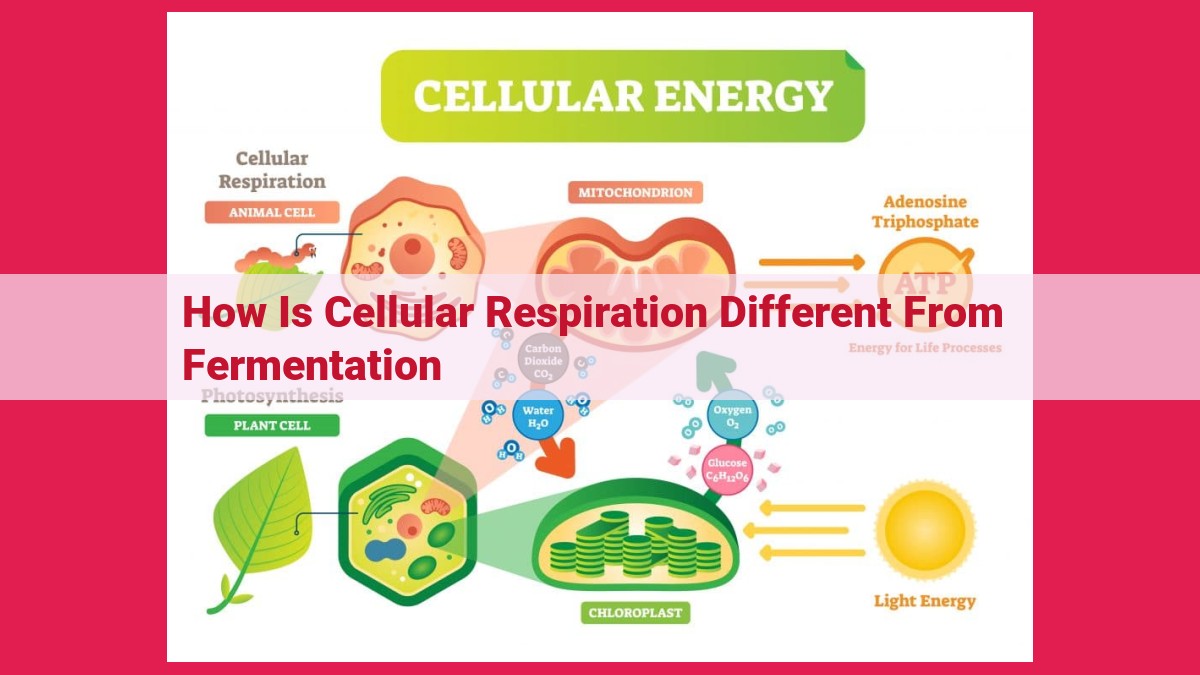
Cellular respiration, requiring oxygen, utilizes the electron transport chain to generate ATP, producing CO2 and water. In contrast, fermentation, occurring in the absence of oxygen, involves pyruvate conversion into lactic acid or ethanol, bypassing the electron transport chain and producing a lower yield of ATP.
Cellular Respiration vs. Fermentation: Unveiling the Secrets of Energy Production
In the vibrant tapestry of life, cells toil tirelessly to generate the energy that fuels our bodies. They rely on two fundamental processes, cellular respiration and fermentation, to extract energy from nutrients.
Cellular respiration is a complex endeavor that occurs within the mitochondria of cells. It is a multi-step process that requires oxygen to break down glucose, a sugar molecule that serves as the primary energy source for cells. As glucose is broken down, chemical energy is released and stored in the form of ATP (adenosine triphosphate), the body’s energy currency.
Fermentation, on the other hand, is a simpler process that occurs in the absence of oxygen. It is常見 found in microorganisms like yeast and bacteria, but also occurs in muscle cells during strenuous exercise. During fermentation, glucose is partially broken down to produce lactic acid or ethanol, releasing less ATP compared to cellular respiration.
Understanding the differences between these two energy production pathways is crucial for comprehending the diverse ways in which organisms harness energy to sustain life. So, let us delve deeper into the intricate world of cellular respiration and fermentation, unraveling the secrets of their energy-generating prowess.
Explain the difference between aerobic respiration, which requires oxygen, and anaerobic fermentation, which does not.
Aerobic Respiration vs. Anaerobic Fermentation: Oxygen’s Vital Role in Energy Production
The human body is an energetic marvel, constantly producing the fuel it needs to function. Two key processes responsible for this energy production are cellular respiration and fermentation. While both processes involve the breakdown of glucose for energy, they differ in a fundamental way: the presence or absence of oxygen.
Aerobic Respiration: Oxygen as the Key
Aerobic respiration is the process by which cells use oxygen to generate energy. It occurs in the presence of oxygen and involves three main steps: glycolysis, the Krebs cycle, and the electron transport chain.
Glycolysis occurs in the cytoplasm and breaks down glucose into two pyruvate molecules. This process generates a small amount of energy in the form of ATP (adenosine triphosphate), the body’s energy currency.
The pyruvate molecules produced in glycolysis enter the Krebs cycle, which takes place in the mitochondria. The Krebs cycle further oxidizes the pyruvate molecules, producing carbon dioxide and more ATP.
The final step, the electron transport chain, is also located in the mitochondria. Here, electrons are passed along a series of proteins, generating a significant amount of ATP through a process called oxidative phosphorylation.
Anaerobic Fermentation: Adapting to Oxygen Scarcity
In contrast, anaerobic fermentation occurs in the absence of oxygen. When the body is deprived of oxygen, such as during intense exercise or in certain tissues, it must rely on fermentation to generate energy.
Anaerobic fermentation also begins with glycolysis, but the process ends there. Instead of entering the Krebs cycle, the pyruvate molecules are converted into either lactic acid or ethanol.
Lactic acid fermentation occurs in muscle cells during strenuous activity. The buildup of lactic acid can lead to muscle fatigue and soreness.
Alcoholic fermentation occurs in yeast cells and some bacteria. It produces ethanol, commonly known as alcohol.
Key Differences
Aerobic respiration requires oxygen and produces a large amount of ATP, while anaerobic fermentation does not require oxygen and generates a smaller amount of ATP.
Aerobic respiration occurs in the mitochondria, while anaerobic fermentation occurs in the cytoplasm.
Aerobic respiration produces carbon dioxide and water as end products, while anaerobic fermentation produces lactic acid (lactic acid fermentation) or ethanol (alcoholic fermentation) as end products.
Cellular Respiration vs. Fermentation: The Power of Oxygen
In the realm of energy production within living organisms, cellular respiration and fermentation play crucial roles. Both processes harness the energy stored in organic molecules to generate fuel for cellular activities. However, they differ fundamentally in their dependency on oxygen.
Aerobic Respiration: The Power of Oxygen
In aerobic respiration, oxygen serves as the electron acceptor. This electron transport chain generates a substantial amount of ATP, the energy currency of the cell. The Krebs cycle, another key component of aerobic respiration, breaks down carbohydrates, releasing energy that further powers the electron transport chain.
Anaerobic Fermentation: Surviving Without Oxygen
In environments where oxygen is scarce, cells resort to anaerobic fermentation to extract energy. This process relies on organic molecules, such as glucose, as electron acceptors. Fermentation produces lactic acid or ethanol as waste products. Although менее efficient than aerobic respiration, fermentation allows cells to survive in oxygen-deprived conditions.
Exploring the Concepts of Oxygen Consumption
Oxygen consumption is the process by which cells utilize oxygen for energy production. Adequate oxygen levels are crucial for maintaining cellular homeostasis and preventing conditions such as:
- Anoxia: A complete lack of oxygen, which can lead to cell death.
- Hypoxia: A condition of inadequate oxygen supply, which can impair cellular functioning and contribute to various health issues.
These concepts highlight the fundamental importance of oxygen in energy production and overall cellular well-being. Understanding the interplay between cellular respiration, fermentation, and oxygen consumption is essential for comprehending the intricate workings of life at the cellular level.
The Electron Transport Chain: Generating Life’s Currency
ATP, the energy currency of life, fuels every cellular function, from muscle contractions to brainpower. But where does this energy come from? Enter the electron transport chain
in partnership with oxidative phosphorylation
. These processes orchestrate a dance of electrons and molecules to synthesize ATP, the fuel that powers our bodies.
Think of the electron transport chain as a conveyor belt, carrying electrons from high-energy molecules like NADH and FADH2. These electrons lose energy as they progress through a series of protein complexes, like stepping down a staircase. This energy loss pumps protons across a membrane, creating an electrochemical gradient.
Imagine a waterfall cascading with protons. This gradient drives the enzyme ATP synthase
, the “electricity generator” of the cell. As protons tumble downhill through the enzyme’s turbine, they cause ATP synthase to rotate, coupling the movement with the synthesis of ATP molecules.
In a nutshell, the electron transport chain extracts energy from electrons, creating a proton gradient that powers ATP synthesis, providing the fuel for all cellular processes. It’s a relentless molecular machinery that keeps the lights of our bodies burning bright.
The Krebs Cycle: Breaking Down Carbohydrates and Generating CO2
The Krebs cycle, also called the citric acid cycle or the Tricarboxylic Acid (TCA) cycle, is a metabolic pathway that plays a crucial role in the breakdown of carbohydrates and the production of CO2. It operates within the mitochondria of eukaryotic cells and is a pivotal stage in cellular respiration.
The Krebs cycle is initiated when pyruvate, a product of glycolysis, enters the mitochondria. Pyruvate is converted into Acetyl CoA, which then combines with oxaloacetate to form citrate, the first intermediate of the cycle. Over the course of multiple enzymatic reactions, citrate undergoes a series of reorganizations, oxidations, and decarboxylations, releasing high-energy electrons and CO2. These reactions yield ATP through substrate-level phosphorylation and generate reducing equivalents (NADH and FADH2) that will later be used in the electron transport chain for additional ATP synthesis.
The Krebs cycle not only breaks down carbohydrates but also plays a central role in the interconversion of various metabolites. It provides precursors for the synthesis of amino acids, lipids, and nucleic acids. This metabolic versatility makes the Krebs cycle an essential hub connecting different pathways within the cell.
The Role of NADH and FADH2 as Electron Carriers
NADH and FADH2, produced during the Krebs cycle and other metabolic reactions, serve as electron carriers in the electron transport chain. These high-energy electrons are passed along a series of protein complexes embedded in the inner mitochondrial membrane. As the electrons move through the chain, their energy is harnessed to pump protons across the membrane, creating an electrochemical gradient. This gradient drives the synthesis of ATP by ATP synthase, the final enzyme in the chain.
Glycolysis: The Initial Stage of Energy Production
As we embark on the metabolic journey of cellular respiration, we encounter the initial stage, glycolysis. Imagine a culinary adventure where glucose, the body’s primary fuel, plays the starring role. In this intricate process, glucose is broken down into a pair of pyruvate molecules, releasing a modest 2 molecules of ATP.
Glycolysis, like a well-choreographed dance, unfolds in three distinct phases:
Phase 1: Activation
The journey begins with glucose activation, where a molecule of ATP donates its high-energy phosphate group to glucose, transforming it into glucose 6-phosphate.
Phase 2: Cleavage
The glucose 6-phosphate, now primed for transformation, undergoes cleavage, splitting into two three-carbon sugars: glyceraldehyde 3-phosphate and dihydroxyacetone phosphate.
Phase 3: Oxidation
In the final phase, oxidation takes center stage. Glyceraldehyde 3-phosphate, oxidized by NAD+, generates two molecules of ATP and two molecules of NADH, a high-energy electron carrier. The dance concludes with dihydroxyacetone phosphate also being oxidized, yielding an additional ATP and **NADH* molecule.
Voila! From a single molecule of glucose, glycolysis extracts 2 pyruvate molecules, 2 ATP molecules, and 2 NADH molecules, setting the stage for the subsequent energy-yielding reactions of cellular respiration or fermentation.
Pyruvate: Fermentation vs. Respiration
In the realm of cellular energy production, pyruvate emerges as a pivotal molecule, its fate determined by the presence or absence of oxygen. When oxygen graces the cellular environment, pyruvate ventures into the Krebs cycle, a labyrinth of biochemical reactions that culminate in the liberation of carbon dioxide (CO2) and the generation of energy-rich molecules.
However, in the absence of oxygen, pyruvate embarks on a different path, a path of anaerobic fermentation. Here, pyruvate is transformed into lactic acid, a by-product of strenuous muscle activity. Alternatively, in certain organisms like yeast, pyruvate is converted into ethanol, the very essence of fermentation that creates your favorite alcoholic beverages.
Thus, pyruvate, like a chameleon, adapts its destiny to the availability of oxygen, ensuring that cells can always extract the energy they need to sustain life.
NADH and FADH2: The Electron Carriers in Cellular Respiration
In the intricate world of cellular respiration, NADH and FADH2 play pivotal roles as electron carriers. These molecules transport electrons through a series of proteins embedded in the inner mitochondrial membrane, forming the electron transport chain.
As glucose is broken down through glycolysis and the Krebs cycle, electrons are stripped away and attached to NAD+ and FAD to form NADH and FADH2. These electron-laden molecules then embark on a journey through the electron transport chain.
The electron transport chain is a series of proteins that transfer electrons from NADH and FADH2 to oxygen. As electrons pass through the chain, their energy is harnessed to pump protons from the mitochondrial matrix to the intermembrane space. This proton gradient creates an electrochemical potential that drives the synthesis of ATP.
NADH and FADH2 donate their electrons at different points in the electron transport chain, releasing varying amounts of energy. NADH donates two electrons and generates more energy, while FADH2 donates only two electrons and produces slightly less energy.
The Significance of NADH and FADH2
The abundance of NADH and FADH2 in a cell is a direct reflection of metabolic activity. High levels of NADH and FADH2 indicate active cellular respiration, while lower levels suggest a switch to anaerobic fermentation.
Furthermore, the ratio of NADH to FADH2 can provide valuable information about cellular conditions. In aerobic conditions, where oxygen is abundant, NADH levels are typically higher than FADH2 levels. However, in anaerobic conditions, FADH2 levels may exceed NADH levels due to the limited electron flow to oxygen.
By understanding the roles of NADH and FADH2 in the electron transport chain, we gain insights into the intricate workings of cellular respiration and how cells generate energy to power their vital functions.
Describe the overall ATP yield in cellular respiration and fermentation, discussing substrate-level phosphorylation and electron transport chain efficiency.
8. ATP Production: The Energy Currency
Each stage of cellular respiration and fermentation produces a specific amount of ATP. Substrate-level phosphorylation occurs when ATP is directly generated from the transfer of a phosphate group from a substrate to ADP. Glycolysis and the Krebs cycle are the two main stages of cellular respiration that produce ATP via substrate-level phosphorylation.
The electron transport chain is a key component of oxidative phosphorylation, where 90% of the ATP in cellular respiration is generated. As electrons pass through the chain, their energy is used to pump protons (H+) across the inner mitochondrial membrane. This creates a proton gradient, which drives the ATP synthase enzyme to produce ATP from ADP and inorganic phosphate (Pi).
ATP Yield:
- Cellular respiration: 30-32 ATP molecules per glucose molecule
- Aerobic respiration: Uses oxygen and produces CO2 and H2O.
- Anaerobic respiration: Does not use oxygen and produces lactic acid or ethanol.
The number of ATP molecules produced varies depending on the efficiency of the electron transport chain and the type of fermentation. For example, lactic acid fermentation produces only 2 ATP molecules per glucose molecule, while alcoholic fermentation produces 2 ATP molecules and 2 molecules of ethanol.
In summary, cellular respiration produces a much higher yield of ATP than fermentation because of the electron transport chain and the final products of CO2 and water. This energy currency is essential for various cellular processes and helps sustain life’s functions.
Cellular Respiration vs. Fermentation: The Tale of Two Energy Pathways
In the bustling city of our cells, energy is the currency that fuels every activity, from pumping ions to synthesizing proteins. Two ingenious energy-generating pathways reside within these cellular powerhouses: cellular respiration and fermentation. Let’s delve into their captivating story, comparing their unique end products.
The End Products: A Tale of Contrast
Cellular Respiration: As oxygen dances through the electron transport chain, a majestic symphony of energy conversion unfolds, culminating in the liberation of carbon dioxide (CO2) and water (H2O) as the ultimate byproducts. These innocent molecules may seem unassuming, but they hold profound significance, signaling the complete oxidation of nutrients for maximum energy extraction.
Fermentation: In the absence of oxygen’s magical presence, fermentation offers a resourceful alternative energy pathway. Pyruvate, a key intermediate in both cellular respiration and fermentation, embarks on a different destiny under these anaerobic conditions. In lactic acid fermentation, pyruvate transforms into lactic acid, providing muscles with energy during strenuous activity. In contrast, alcoholic fermentation, a process embraced by yeast, converts pyruvate into ethanol, the intoxicating spirit that enchants many a celebration.
The End Product Divide: Unraveling the Differences
The contrasting end products of cellular respiration and fermentation reflect their distinct mechanisms and energy yields. Cellular respiration harnesses the full power of oxygen to maximize energy extraction, culminating in the complete breakdown of nutrients and the release of CO2 and H2O. This pathway is a highly efficient energy producer, yielding a whopping 36-38 molecules of ATP per glucose molecule.
Fermentation operates on a more modest scale, producing a mere 2 molecules of ATP per glucose molecule. Its anaerobic nature limits the extent of nutrient breakdown, resulting in the accumulation of lactic acid or ethanol. These end products may seem like waste, but they serve a vital role in certain cellular contexts. Lactic acid can fuel muscles during intense exercise, while ethanol plays a social and industrial role in beverages and biofuels.
The Interplay of Oxygen: A Critical Distinction
The presence or absence of oxygen dictates the fate of pyruvate, the branching point between cellular respiration and fermentation. In the oxygen-saturated realm of aerobes, pyruvate happily enters the Krebs cycle, a metabolic maze that culminates in the electron transport chain. This pathway leads to the production of CO2 and H2O along with an abundance of ATP.
Conversely, when oxygen becomes scarce, cells switch gears to fermentation. Pyruvate is funneled into alternative pathways, yielding either lactic acid or ethanol as end products. This anaerobic process still generates ATP, but to a lesser extent than cellular respiration.
Cellular respiration and fermentation, despite their distinct end products and energy yields, share a common goal: to generate ATP, the cellular energy currency. Their complementary roles ensure that cells can adapt to diverse environmental conditions, whether in the oxygen-rich atmosphere or in the anaerobic depths. By understanding these pathways, we gain a deeper appreciation for the intricate dance of life within our cellular powerhouses.
Cellular Respiration vs. Fermentation: An Energy Tale
Begin with a hook:
In the bustling city of our bodies, there’s a constant energy crisis going on. Our cells, the bustling metropolis’s tiny inhabitants, require a steady supply of energy to power their activities. That’s where cellular respiration and fermentation step in, like two valiant knights vying to provide this vital fuel.
Subheading 1: The Mighty Cellular Respiration
Cellular respiration is the aerobic champ, the oxygen-loving process that harnesses the power of oxygen to generate energy. Like a well-oiled machine, it breaks down glucose, the body’s primary energy source, into carbon dioxide and water.
Subheading 2: The Resilient Fermentation
Fermentation, on the other hand, is the anaerobic underdog, the oxygen-independent way to produce energy. When oxygen is scarce, fermentation steps up to the plate, converting glucose into lactic acid or ethanol.
Subheading 3: The Energy Production Powerhouses
Both cellular respiration and fermentation are geared towards ATP production, the body’s universal energy currency. Cellular respiration is the reigning champion, producing a whopping 36 ATP molecules for every glucose molecule. Fermentation, while not as efficient, still manages to generate 2 ATP molecules.
Subheading 4: The End Product Divide
The tale unfolds with a significant difference in their end products. Cellular respiration releases carbon dioxide and water, while fermentation leaves behind lactic acid or ethanol. These end products reflect the different paths taken by these energy-producing processes.