Cellular Respiration: A Guide To Metabolic Diversity Across Organisms
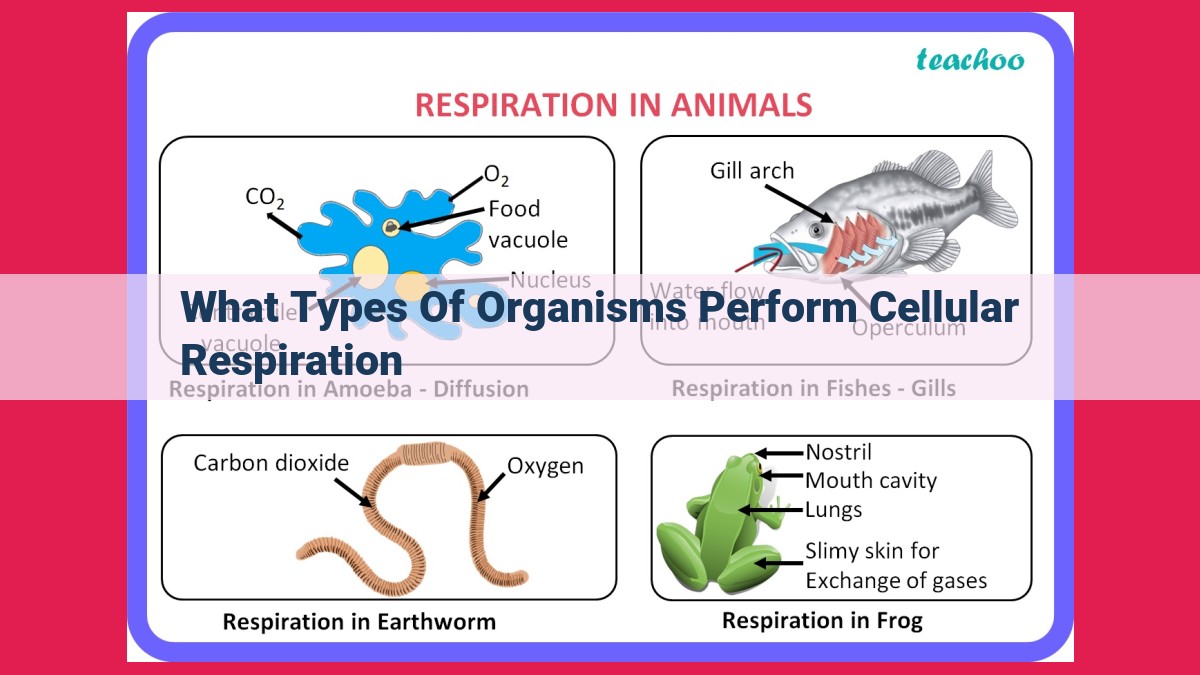
Cellular respiration, a vital process for energy production, is performed by diverse organisms. From bacteria to animals, these organisms fall into various categories based on their respiratory requirements: aerobic, anaerobic, facultative anaerobes, obligate aerobes, and obligate anaerobes. Aerobic organisms rely on oxygen, while anaerobic organisms don’t. Facultative anaerobes can switch between aerobic and anaerobic respiration, while obligate aerobes solely use oxygen and obligate anaerobes strictly avoid it. These variations in respiratory mechanisms reflect the metabolic diversity and adaptability of organisms in their respective environments.
Cellular Respiration: A Tale of Life’s Energetic Dance
What is Cellular Respiration?
Picture a bustling city, where every cell is a tiny universe, abuzz with activity. At the very heart of this energetic metropolis lies cellular respiration, the life-sustaining process that converts the sugars we eat into the driving force of life: energy.
Who Participates in this Dance?
Cellular respiration is not limited to a select few; it’s a universal language spoken by all living organisms. From the tiniest bacteria to the majestic blue whale, every living creature relies on cellular respiration to power their existence.
Classifying the Players:
Just as there are different languages spoken in the world, there are also different types of cellular respiration. Organisms can be classified based on their respiratory preferences:
-
Aerobic Organisms: These are the oxygen-loving crowd, who thrive in the presence of this vital gas. They utilize a highly efficient process known as aerobic respiration, which harnesses the power of oxygen to generate massive amounts of energy.
-
Anaerobic Organisms: In contrast to their oxygen-dependent counterparts, anaerobic organisms can boogie even without oxygen. They employ a less efficient but still effective process called anaerobic respiration, which produces energy through fermentation.
-
Facultative Anaerobes: These clever creatures are the ultimate opportunists, capable of switching between aerobic and anaerobic respiration depending on the availability of oxygen. They’re like the masters of both worlds, always ready to adapt and survive.
-
Obligate Aerobes: These are the oxygen purists, who strictly rely on aerobic respiration for their energetic needs. They have no Plan B, so oxygen is their lifeblood.
-
Obligate Anaerobes: On the opposite end of the spectrum, we have the oxygen-intolerant crowd. These organisms can’t tolerate the presence of oxygen and perform anaerobic respiration exclusively.
Aerobic Organisms: Oxygen-Dependent Respiration
- Explain the principles and process of aerobic respiration.
- Describe the role of mitochondria in aerobic respiration.
- Highlight the production of ATP through oxidative phosphorylation.
Aerobic Organisms: The Oxygen-Dependent Breath of Life
In the vibrant tapestry of life, organisms exhibit an extraordinary diversity in their metabolic processes. Among them, aerobic organisms stand out as masters of oxygen-dependent respiration, a process that unlocks the energy stored within organic molecules to fuel their cellular activities.
Aerobic respiration unfolds within the mitochondria, the powerhouses of eukaryotic cells. Here, a complex symphony of biochemical reactions, orchestrated by enzymes, transforms glucose, a simple sugar, into ATP (adenosine triphosphate), the universal currency of energy in living cells.
The intricacies of aerobic respiration begin with glycolysis, a preparatory phase that occurs in the cytoplasm outside the mitochondria. Glycolysis breaks down glucose into smaller molecules, yielding a paltry amount of ATP. The real energy harvest takes place within the mitochondria, where the pyruvate molecules produced in glycolysis undergo further processing.
Within the Krebs cycle, also known as the citric acid cycle, pyruvate is oxidized, releasing carbon dioxide as a waste product and high-energy electrons. These electrons are captured by electron carriers and passed through an electron transport chain embedded in the inner mitochondrial membrane.
As electrons cascade down the transport chain, their energy is harnessed to pump protons across the membrane, creating an electrochemical gradient. This gradient drives the synthesis of ATP through oxidative phosphorylation, the final and most efficient stage of aerobic respiration. The influx of protons back into the mitochondrial matrix through ATP synthase enzymes generates ATP, replenishing the cellular energy reserves.
Thus, aerobic organisms, endowed with an intimate relationship with oxygen, have evolved an efficient and intricate process to extract energy from organic molecules, sustaining their metabolic needs and fueling the complexities of life.
Anaerobic Organisms: Thriving Without Oxygen
In the realm of life, some organisms possess an extraordinary ability to survive and produce energy in the absence of oxygen. These anaerobic organisms have evolved unique mechanisms to harness energy from glucose without the involvement of this vital gas.
Principles of Anaerobic Respiration
Unlike aerobic organisms that rely on oxygen for cellular respiration, anaerobic organisms employ a different pathway known as anaerobic respiration. This process occurs in the cytoplasm of the cells, where glucose is broken down into smaller molecules. The key distinction from aerobic respiration is the absence of oxygen as an electron acceptor.
Role of the Cytoplasm
The cytoplasm plays a central role in anaerobic respiration. Here, a series of chemical reactions take place, each catalyzed by specific enzymes. These reactions break down glucose into pyruvate, a three-carbon compound. In the absence of oxygen, pyruvate is further metabolized through a process called fermentation.
Production of ATP Through Fermentation
Fermentation is the final step of anaerobic respiration, where pyruvate is converted into various end products. One common pathway is lactic acid fermentation, in which pyruvate is converted into lactic acid. This process is utilized by bacteria and some muscle cells during intense exercise.
Another type of fermentation is alcoholic fermentation, in which pyruvate is converted into ethanol (alcohol) and carbon dioxide. This process is carried out by yeast and is responsible for the production of alcoholic beverages.
Regardless of the end product, fermentation generates a small amount of energy in the form of ATP. This energy is crucial for the survival and growth of anaerobic organisms in oxygen-depleted environments.
Facultative Anaerobes: The Versatile Respirometers
Amidst the diverse cast of organisms that grace our planet, facultative anaerobes stand out with their remarkable ability to adapt their respiration to the whims of their environment. These metabolic magicians can switch between aerobic respiration, which requires oxygen, and anaerobic respiration, which makes do without it.
Oxygen-Dependent Aerobic Respiration
When oxygen is readily available, facultative anaerobes embrace the efficiency of aerobic respiration. This powerhouse process takes place within the specialized organelles known as mitochondria, where oxygen acts as the final electron acceptor in the electron transport chain. Through this cascade of electron transfers, a staggering amount of ATP (the cellular currency of energy) is generated via oxidative phosphorylation.
Oxygen-Independent Anaerobic Respiration
When oxygen becomes scarce, facultative anaerobes seamlessly transition to anaerobic respiration. The workhorse for this process is the cytoplasm, where glucose is partially broken down through fermentation pathways. While less efficient than aerobic respiration, fermentation still yields a modest amount of ATP to keep the cell ticking over.
Metabolic Versatility at Play
The metabolic flexibility of facultative anaerobes is remarkable. When oxygen is plentiful, they prioritize aerobic respiration, maximizing their ATP production. When oxygen is scarce, they swiftly switch to anaerobic respiration, ensuring their survival.
Examples of Facultative Anaerobes
Yeasts are classic examples of facultative anaerobes. In the presence of oxygen, they thrive on aerobic respiration, fermenting sugars to produce ethanol and carbon dioxide. Bacteria such as E. coli also exhibit facultative anaerobic respiration, switching to fermentation when oxygen is depleted.
Facultative anaerobes are masters of metabolic adaptation, capable of harnessing energy from a variety of sources. Their versatile respiration allows them to thrive in a wide range of environments, from oxygen-rich to oxygen-poor. These remarkable organisms are a testament to the diversity and ingenuity of life on Earth.
Obligate Aerobes: Essential Oxygen for Life
In the vast tapestry of living organisms, obligate aerobes stand as unique entities, their existence intricately intertwined with the life-giving gas, oxygen. Unlike other organisms that possess versatile respiratory pathways, obligate aerobes have evolved to depend solely on oxygen for their metabolic needs.
These organisms possess a unique respiratory mechanism that is exclusively confined to the mitochondria, the energy powerhouses of cells. This exclusive location allows for efficient oxidative phosphorylation, a process that harnesses the energy stored in oxygen to produce the vital molecule ATP.
ATP serves as the universal energy currency for all cells, powering countless cellular processes. Obligate aerobes rely exclusively on oxidative phosphorylation for ATP production, making them utterly dependent on oxygen. Without a steady supply of oxygen, these organisms would perish, their metabolic machinery grinding to a halt.
Examples of obligate aerobes include the majority of vertebrates, including humans, as well as many bacteria and fungi. These organisms populate diverse environments, from the depths of the ocean to the highest mountain peaks, wherever oxygen is available in sufficient quantities.
In ecosystems, obligate aerobes play a crucial role in nutrient cycling and decomposition processes. They are responsible for breaking down organic matter, releasing essential nutrients back into the environment. Their presence is a testament to the indispensable role of oxygen in sustaining life on Earth.
Obligate Anaerobes: The Oxygen-Intolerant Respirers
In the realm of cellular respiration, there exist a unique group of organisms known as obligate anaerobes. Unlike their oxygen-dependent counterparts, these microorganisms possess an unusual aversion to the very gas that sustains most life forms. Obligate anaerobes meticulously avoid exposure to oxygen, as it instigates a toxic reaction that puts their metabolic processes at risk.
Obligate anaerobes have evolved to perform respiration in a completely oxygen-free environment. This exclusive preference for anaerobic conditions necessitates strategic adaptation. Unlike aerobic organisms, which utilize specialized organelles called mitochondria for respiration, obligate anaerobes conduct their respiratory processes within the boundless expanse of their cytoplasm.
The absence of mitochondria does not hinder obligate anaerobes in producing energy. They have developed intricate pathways to generate ATP, the molecule that powers cellular activities. Obligate anaerobes accomplish this feat through a process known as fermentation.
Fermentation is a unique form of respiration that occurs in the absence of oxygen. During fermentation, glucose, a sugar molecule, is broken down into various products, including lactic acid or alcohol. This breakdown yields a modest amount of ATP, just enough to fuel cellular processes. While fermentation is less efficient than oxidative phosphorylation, the mitochondrial respiration process used by aerobic organisms, it suffices for the energy needs of obligate anaerobes.
So, there you have it—the obligate anaerobes, fascinating microorganisms that flourish in oxygen-starved environments. Their exclusive reliance on fermentation and aversion to oxygen make them unique players in the vast tapestry of life on Earth.