Understanding Cellular Respiration: The Powerhouse Of Energy Production
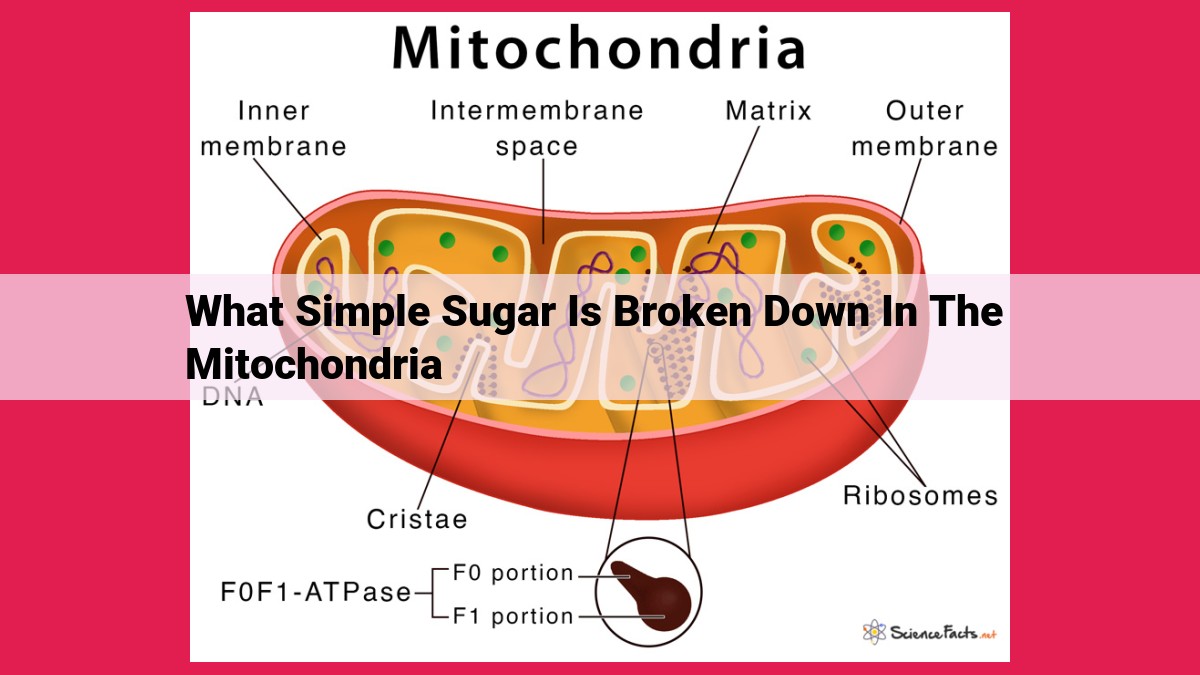
In the mitochondria, a pivotal organelle for energy production, the simple sugar glucose is broken down through a series of chemical reactions known as cellular respiration. The initial phase, glycolysis, initiates this process by splitting glucose into two molecules of pyruvate. Pyruvate then enters the citric acid cycle, a central metabolic pathway that generates energy-rich molecules such as ATP, the primary energy currency of cells. The electron transport chain, a critical component in this process, transfers electrons along a series of protein complexes, releasing energy used to produce ATP through oxidative phosphorylation.
Cellular Respiration: An Overview
Cellular respiration is the process by which cells generate energy to power their life-sustaining activities. It’s the cornerstone of metabolism, the intricate network of chemical reactions that occur within living organisms. Without cellular respiration, cells would quickly run out of energy and cease to function.
At the heart of cellular respiration lies glucose, a sugar molecule that serves as the main fuel for most cells. Through a series of complex chemical reactions, glucose is broken down to release energy, which is then stored in the form of adenosine triphosphate (ATP). ATP acts as the universal energy currency of cells, powering everything from muscle contractions to nerve impulses.
Cellular respiration is an essential process that sustains life on Earth. It provides the fuel that powers cellular activities, enabling cells to grow, reproduce, and respond to their environment. Understanding cellular respiration is crucial for appreciating the intricate workings of living organisms.
Glycolysis: Breaking Down Glucose
- Describe the first step of cellular respiration, where glucose is broken down.
Glycolysis: Breaking Down Glucose, the First Step in the Energy-Generating Process
Glycolysis, the initial stage of cellular respiration, plays a crucial role in providing energy for cells. It begins the journey of glucose, the body’s main source of energy, as it undergoes a series of chemical reactions to release its stored energy.
The Breakdown Process
Glycolysis occurs in the cytoplasm of the cell and serves as the gateway for glucose metabolism. This complex process involves 10 enzymatic steps that systematically break down glucose into smaller molecules, primarily pyruvate. Along with pyruvate, glycolysis also generates ATP (adenosine triphosphate), the universal energy currency of cells.
The Energy Harvest
During glycolysis, glucose, a six-carbon sugar, is broken down into two three-carbon molecules of pyruvate. This process releases a net of 2 molecules of ATP per glucose molecule. Additionally, 2 molecules of NADH, a high-energy electron carrier, are produced. These NADH molecules serve as vital intermediates in the subsequent stages of cellular respiration, carrying electrons that will ultimately contribute to ATP generation.
Significance of Glycolysis
Glycolysis is a crucial step in cellular respiration, providing initial energy in the form of ATP and NADH. It acts as the foundation for further energy production through the subsequent processes of the citric acid cycle and oxidative phosphorylation. Without glycolysis, cells would be deprived of the energy they need to perform their essential functions, leading to compromised cellular processes and ultimately cell death.
Pyruvate: The Versatile Intermediate in Glucose Metabolism
In the intricate tapestry of cellular respiration, pyruvate emerges as a pivotal intermediate, a versatile molecule that plays a crucial role in glucose metabolism. As the product of glycolysis, the initial stage of cellular respiration, pyruvate holds the key to unlocking the energy stored within the glucose molecule.
This versatile intermediate can embark on multiple metabolic pathways, each leading to a distinct outcome. Its fate is dictated by the cellular context and the organism’s energy needs. For instance, in the presence of oxygen, pyruvate undergoes oxidative phosphorylation, the process that generates the majority of cellular energy.
When oxygen is scarce, pyruvate takes an alternative route, fermenting into lactic acid or ethanol, providing a temporary energy supply under anaerobic conditions. Pyruvate’s flexibility showcases its adaptability in meeting the varying demands of energy production.
Furthermore, pyruvate serves as a precursor for the synthesis of several essential biomolecules, including amino acids, fatty acids, and nucleotides. Its involvement in these biosynthetic pathways highlights its significance beyond energy production.
As a gatekeeper of glucose metabolism, pyruvate regulates the flow of energy and building blocks throughout the cell. Its versatility and pivotal role underscore its importance in sustaining cellular functions and maintaining metabolic homeostasis.
Acetyl-CoA: Fueling the Citric Acid Cycle
The Citric Acid Cycle’s Powerhouse
At the heart of cellular respiration lies the citric acid cycle, a metabolic pathway that transforms glucose into energy-rich molecules. But this cycle wouldn’t be possible without a crucial player: acetyl-CoA. Derived from pyruvate, a byproduct of glycolysis, acetyl-CoA serves as the fuel for the citric acid cycle.
Acetyl-CoA’s Journey
Acetyl-CoA enters the citric acid cycle by combining with a four-carbon molecule known as oxaloacetate. This union initiates a series of nine chemical reactions that generate energy-rich molecules, including ATP and NADH.
The Citric Acid Cycle’s Role
The citric acid cycle operates like a merry-go-round, continuously regenerating oxaloacetate to accept more acetyl-CoA molecules. Each turn of the cycle oxidizes acetyl-CoA, releasing carbon dioxide as a byproduct. Simultaneously, energy-carrier molecules like NADH and FADH2 are produced.
NADH and FADH2: The Energy Couriers
The energy stored in NADH and FADH2 is harvested in the electron transport chain, another critical component of cellular respiration. These molecules transfer electrons along a series of proteins within the mitochondrial membranes, releasing energy used to pump protons across the membrane.
ATP: The Energy Currency
The proton gradient created by the electron transport chain drives ATP synthase, an enzyme that synthesizes ATP from ADP. ATP, the currency of cellular energy, powers countless cellular processes, from muscle contraction to nerve transmission.
The Acetyl-CoA Link
Without acetyl-CoA, the citric acid cycle would grind to a halt, disrupting the entire process of cellular respiration. It’s the acetyl-CoA that fuels the citric acid cycle, providing the energy-rich molecules that sustain life’s processes.
The Citric Acid Cycle: Energy Generation Hub
Step into the bustling metropolis of the cell, where countless biochemical reactions occur. Among them shines the Citric Acid Cycle, a pivotal energy generator that powers our daily functions. This labyrinth of chemical transformations holds the secret to unlocking the energy stored within glucose.
As Acetyl-CoA enters this metabolic maze, it joins forces with a four-carbon molecule called oxaloacetate, forming citrate. A series of nine enzymatic reactions ensue, culminating in the regeneration of oxaloacetate, ready to start the cycle anew.
But don’t be fooled by its cyclic nature. With each turn of the cycle, high-energy molecules emerge. Energy-rich electrons are captured and transferred to coenzymes, fueling the electron transport chain and ultimately resulting in the creation of precious ATP.
Like a tireless worker, the citric acid cycle tirelessly converts acetyl groups into carbon dioxide. As it does so, it harvests energy that drives the production of NADH (nicotinamide adenine dinucleotide) and FADH2 (flavin adenine dinucleotide), which serve as the spark plugs of the electron transport chain.
Moreover, the citric acid cycle provides building blocks for other essential cellular processes. It generates precursors for amino acids, nucleotides, and lipids—the fundamental components of life.
In essence, the Citric Acid Cycle is the beating heart of our cells. It tirelessly generates the power that allows us to move, think, and thrive. By unraveling the secrets of this metabolic marvel, we gain a deeper appreciation for the intricate workings of our bodies and the extraordinary dance of life that unfolds within them.
Electron Transport Chain: Passing Electrons
- Explain the role of the electron transport chain in transferring electrons for energy production.
The Electron Transport Chain: Powerhouse of Energy Production
After pyruvate is broken down, its energy is captured and carried by a molecule called acetyl-CoA. This molecule serves as the fuel for the next stage of cellular respiration: the citric acid cycle.
The citric acid cycle takes place in the mitochondria, the powerhouse of the cell. Here, acetyl-CoA joins with a series of carrier molecules and undergoes a series of chemical transformations. As the molecules pass through the cycle, they release energy by transferring electrons to specialized proteins called the electron transport chain.
The electron transport chain is a series of protein complexes embedded in the mitochondrial membrane. Like a conveyor belt, these complexes pass electrons from one to another, releasing energy with each transfer. This energy is used to pump protons across the membrane, creating a concentration gradient.
The protons eventually flow back into the mitochondria through a channel called ATP synthase, driving the synthesis of ATP. ATP is the cell’s energy currency, and this process of oxidative phosphorylation allows cells to harness the energy released from the electron transport chain to produce the fuel they need to power their activities.
In essence, the electron transport chain acts as an electron freeway, carrying electrons through a series of energy-releasing reactions. These reactions ultimately generate the ATP that fuels the myriad of cellular processes essential for life.
Oxidative Phosphorylation: Generating ATP
- Discuss how the energy released from electron transfer is used to produce ATP, the cell’s energy currency.
Oxidative Phosphorylation: Generating the Cell’s Energy Currency
In the thrilling saga of cellular respiration, the electron transport chain plays a pivotal role as a colossal electron conveyor belt. As electrons leapfrog through this chain, their kinetic energy is ingeniously harnessed to pump protons across a membrane, creating a proton gradient. This gradient stores a wealth of potential energy, like a coiled spring awaiting release.
Enter oxidative phosphorylation, the grand finale of this cellular symphony. This process harnesses the pent-up energy of the proton gradient to generate the lifeblood of cells: ATP. Picture a molecular turbine, where the downhill flow of protons drives the synthesis of this precious molecule. With each molecule of ATP formed, a burst of energy is unleashed, ready to fuel the myriad activities of life.
ATP, the powerhouse of cells, is the universal energy currency that fuels a dazzling array of cellular processes, from muscle contractions to nerve impulses. It’s like the gold standard of cellular energy, powering essential functions that keep us alive and kicking.
In the realm of cellular respiration, oxidative phosphorylation stands as the ultimate energy generator, transforming the raw fuel of glucose into the life-sustaining currency of ATP. It’s a testament to the remarkable symphony of life, where complex biochemical pathways dance in perfect harmony to provide the energy that fuels our every breath and heartbeat.
ATP: The Powerhouse of Cells
In the bustling metropolis of your cells, there exists a bustling energy hub – adenosine triphosphate (ATP), the lifeblood of all cellular activities. ATP is a small, yet mighty, molecule that acts as the primary energy currency powering every aspect of cell life.
Just like a bustling city needs electricity to function, your cells rely heavily on ATP for their daily operations. From the tiniest flicker of a nerve impulse to the mighty contraction of your muscles, each cellular function consumes ATP. This molecule is the workhorse that fuels every process, ensuring your cells thrive and perform their vital tasks seamlessly.
ATP consists of a molecule of adenosine and three phosphate groups linked together. The bond between the last two phosphate groups is particularly energy-rich, making ATP the ideal energy carrier. When the cell needs energy, it breaks this bond, releasing a burst of energy that can be harnessed to drive cellular processes.
The role of ATP is not limited to cellular functions; it also plays a crucial part in our overall health and well-being. The constant turnover of ATP in our cells is essential for maintaining proper body temperature, supporting tissue growth, and repairing damaged cells. Without ATP, our bodies would quickly succumb to exhaustion and disrepair.
Understanding the significance of ATP is like understanding the heart of life itself. It’s the fuel that powers our cells, the spark that ignites our actions, and the driving force behind our very existence. As we delve deeper into the world of cellular respiration, we’ll continue to appreciate the remarkable role of ATP in keeping us alive and thriving.