Cellular Respiration: Unlocking The Energy Currency Of Cells
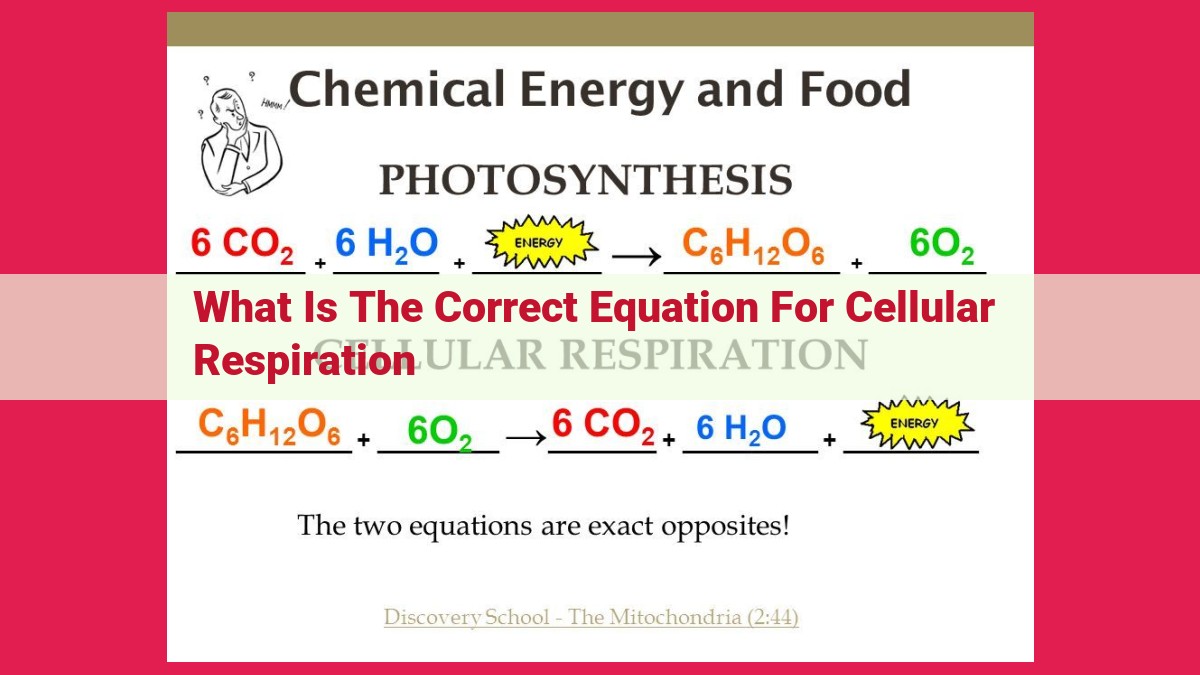
The equation for cellular respiration is: C6H12O6 + 6O2 → 6CO2 + 6H2O + energy (ATP). This process involves the breakdown of glucose (C6H12O6) in the presence of oxygen (O2) to produce carbon dioxide (CO2), water (H2O), and energy in the form of ATP (adenosine triphosphate). ATP is the primary energy currency of cells and is used to power various cellular processes.
Understanding Cellular Respiration: The Foundation of Life’s Energy
At the heart of every living organism lies a complex dance of chemical reactions known as cellular respiration. It’s the process by which cells convert glucose, the energy currency of life, into ATP, the molecule that powers all cellular activities. Without cellular respiration, our bodies would grind to a halt, unable to move, think, or even breathe.
Importance of Cellular Respiration
Cellular respiration is essential for life because it:
- Provides energy: ATP fuels every aspect of cellular function, from muscle contraction to DNA replication.
- Maintains cellular homeostasis: Cells constantly adjust their internal environment to maintain optimal conditions for survival. Cellular respiration helps regulate pH, temperature, and nutrient availability.
- Detoxifies the body: Certain byproducts of metabolism are toxic to cells. Cellular respiration helps eliminate these substances.
Cellular Respiration: The Story of Energy for Life
Cellular respiration, the process by which living organisms convert food into energy, is like a beautiful dance with intricate steps. It’s a journey through three main stages that unlock the power hidden within the molecules we consume.
Glycolysis: The Opening Act
The first stage, glycolysis, takes place in the cytoplasm of cells. Here, glucose, the simple sugar we get from food, is broken down into two pyruvate molecules. This breakdown releases a small amount of energy, captured in the form of two ATP (adenosine triphosphate) molecules. ATP, the energy currency of cells, fuels all cellular processes.
Krebs Cycle: The Dance in the Mitochondria
Pyruvate molecules, carrying their energy-rich carbon atoms, enter the mitochondria, the cell’s powerhouses. In the Krebs cycle, a series of chemical reactions slowly oxidize pyruvate, releasing more energy and reducing power (in the form of NADH and FADH2).
Oxidative Phosphorylation: The Final Act
The climax of cellular respiration, oxidative phosphorylation, occurs on the inner mitochondrial membrane. Here, electrons from NADH and FADH2 are passed through a series of electron carriers, creating a proton gradient across the membrane. The gradient drives ATP synthesis through a process called chemiosmosis, producing a significant amount of energy (up to 32 ATP molecules per glucose).
Cellular respiration, the intricate choreography of glycolysis, Krebs cycle, and oxidative phosphorylation, is the essential life force that drives every living cell. From the simplest bacteria to the most complex humans, this process provides the energy for growth, metabolism, movement, and all the wonders of life. Understanding cellular respiration is key to unraveling the mysteries of our own biology and the intricate workings of the natural world.
Aerobic Respiration
- Explain the process of aerobic respiration, which requires oxygen as an electron acceptor.
Aerobic Respiration: Embracing Oxygen for Energy Production
Aerobic respiration is a fundamental process that enables countless living organisms to harness energy from glucose for their survival and growth. It’s a biochemical symphony that requires the presence of oxygen as an electron acceptor. Unlike its anaerobic counterpart, aerobic respiration operates with far greater efficiency, yielding a significantly larger energy harvest in the form of ATP.
The intricate dance of aerobic respiration unfolds within the powerhouses of cells, the mitochondria. Here, glucose, the primary energy currency of life, enters the stage. Through a series of carefully choreographed reactions, glucose is broken down into smaller molecules, releasing energy that is captured and stored as ATP.
During this process, oxygen plays a crucial role as the final electron acceptor. Electron carriers, such as NADH and FADH2, pass on their electrons to a chain of protein complexes known as the electron transport chain. As electrons cascade through this chain, they release energy that is harnessed to pump protons across the mitochondrial inner membrane.
This proton gradient, a buildup of protons on one side of the membrane, creates an electrochemical force that drives the final step of aerobic respiration: oxidative phosphorylation. Here, the enzyme ATP synthase uses the proton gradient as a source of energy to synthesize ATP, the universal energy molecule of the cell.
The result of aerobic respiration is a bountiful harvest of ATP, providing cells with the fuel they need to power their activities. From muscle contraction and nerve transmission to organ function and cellular growth, the energy generated by aerobic respiration forms the lifeblood of countless biological processes.
By embracing oxygen as an electron acceptor, aerobic respiration unlocks a treasure trove of energy that sustains the vibrant symphony of life. It is a testament to the intricate and elegant workings of the natural world, enabling organisms to thrive and flourish in an oxygen-rich environment.
Anaerobic Respiration: Surviving Without Oxygen
In the depths of our biological realm, cellular respiration reigns supreme, the lifeblood of every living organism. But what happens when the air we breathe, the lifeline of aerobic respiration, is scarce? Enter anaerobic respiration, a fascinating adaptation that allows life to thrive even in oxygen-deprived environments.
Anaerobic respiration, as its name suggests, takes place without oxygen‘s presence. In this unique process, cells resort to an alternative electron acceptor, a molecule other than oxygen, to complete the respiratory chain. This adaptation enables organisms to squeeze every last bit of energy from glucose, even in the absence of the vital gas.
Fermentation: The Essence of Anaerobic Respiration
At the heart of anaerobic respiration lies fermentation, a series of biochemical reactions that occur in the cytoplasm, the fluid-filled interior of cells. During fermentation, glucose, the primary energy source for cells, is broken down into various end products, depending on the specific organism and the fermentation pathway it employs.
Two Major Fermentation Pathways
Lactic acid fermentation is a common type of fermentation found in muscle cells during strenuous exercise. When oxygen supply to these cells is limited, they rely on anaerobic respiration to produce energy. The end product of this pathway is lactic acid, which accumulates in muscles, causing the familiar burning sensation we experience during intense workouts.
Another prevalent fermentation pathway is alcoholic fermentation, utilized by microorganisms such as yeast. In this process, glucose is converted into ethanol (alcohol) and carbon dioxide. This fermentation is the basis of alcoholic beverage production and is also employed in bread-making, where the released carbon dioxide causes dough to rise.
Significance of Anaerobic Respiration
Anaerobic respiration is a remarkable adaptation that allows organisms to survive and flourish in environments with low or fluctuating oxygen levels. It enables us to push through challenging workouts, and it also plays a crucial role in the food industry, providing us with delectable treats like bread and alcoholic beverages.
By understanding anaerobic respiration, we gain a deeper appreciation for the diversity and resilience of life on our planet. It reminds us that even in the face of adversity, living organisms have evolved intricate strategies to harness energy and thrive.
Glycolysis
- Outline the first stage of both aerobic and anaerobic respiration, where glucose is broken down into pyruvate.
Glycolysis: The First Act of Cellular Respiration’s Energy Symphony
In the grand opera of cellular respiration, glycolysis serves as the captivating opening act, setting the stage for the interplay of molecules that will culminate in the creation of life-sustaining energy. This intricate process is performed at the cellular level by all living organisms, from the tiniest bacteria to the vast complexity of our human bodies.
The Breakdown of Glucose: Breaking Down the Sweet Symphony
Glycolysis takes our body’s primary fuel, glucose, and initiates its conversion into two molecules of pyruvate. This transformation occurs in a series of intricate chemical reactions within the cell’s cytoplasm. Each reaction is orchestrated by specific enzymes, the tireless workers of our biological machinery.
A Journey of Ten Steps: The Path of Glucose Conversion
The journey of glycolysis can be likened to a well-choreographed dance involving ten distinct steps. Each step involves the meticulous breakdown of glucose, accompanied by the transfer of electrons and the generation of two high-energy molecules:
- NADH and FADH2, the energy-storing molecules that will play a pivotal role in later stages of cellular respiration.
The Net Energy Gain: Reaping the Rewards of Breakdown
As glycolysis concludes, the dance of chemical reactions has yielded a net gain of two ATP molecules. ATP, the universal energy currency of cells, will be used to power cellular functions, from muscle contractions to the synthesis of life-essential molecules.
Energy for All: Aerobic and Anaerobic Respiration
Glycolysis serves as the cornerstone of both aerobic and anaerobic respiration. Aerobic respiration, the more efficient energy-generating pathway, requires the presence of oxygen. Anaerobic respiration, on the other hand, proceeds without oxygen and relies on fermentation processes. The choice between these pathways depends on the availability of oxygen within the cellular environment.
A Window into Metabolism: Understanding Cellular Respiration
Glycolysis stands as a testament to the incredible complexity of life’s processes. It is a foundational step in the energy-generating journey of cellular respiration, providing essential energy to sustain the countless activities that maintain our existence. By delving into this intricate dance of molecules, we gain a deeper appreciation for the wonders of life and the intricate machinery that powers our very being.
The Krebs Cycle: Unlocking Energy’s Secrets
In the heart of our cells, where life’s dance unfolds, there exists a remarkable energy-producing pathway known as cellular respiration. After glycolysis, the first stage that breaks down glucose, the journey continues with the Krebs cycle.
This enigmatic cycle, named after its discoverer, Sir Hans Krebs, takes place within the mitochondria, the powerhouse of our cells. Here, a molecule of acetyl-CoA, a two-carbon fragment, embarks on a whirlwind of chemical reactions that release significant amounts of ATP, the energy currency of life.
As acetyl-CoA enters the cycle, it combines with a four-carbon molecule called oxaloacetate to form a six-carbon compound called citrate. citrate then undergoes a series of transformations, releasing carbon dioxide and reducing electron carriers. These carriers, NADH and FADH2, will later be used in the final stage of cellular respiration, oxidative phosphorylation, to generate even more ATP.
After completing one full turn of the Krebs cycle, oxaloacetate is regenerated, ready to accept another acetyl-CoA and start the cycle anew. In total, each molecule of glucose entering cellular respiration yields two molecules of acetyl-CoA, leading to two turns of the Krebs cycle and the production of up to 36 ATP molecules.
The Krebs cycle is a critical component of cellular respiration, providing the bulk of the energy that powers our bodies. Without this intricate dance of chemical reactions, life as we know it would cease to exist.
Electron Transport Chain: The Energy Generator of Cells
In the realm of cellular respiration, there exists a cascade of protein complexes known as the electron transport chain (ETC). These intricate structures reside within the mitochondrial membrane and play a pivotal role in extracting the stored energy from nutrients and converting it into the cellular fuel, adenosine triphosphate (ATP).
The ETC operates akin to a conveyor belt, meticulously passing electrons from molecule to molecule. As these electrons traverse the chain, their energy is harnessed to pump protons across the mitochondrial inner membrane, establishing a proton gradient. This gradient represents a reservoir of potential energy, awaiting its release to power the final stage of cellular respiration – oxidative phosphorylation.
Imagine the ETC as a series of protein complexes, each a distinct entity with a specific task. The first complex, NADH dehydrogenase, receives electrons from NADH, the electron carrier generated during glycolysis and the Krebs cycle. As these electrons flow through the complex, they release energy that drives the pumping of protons across the membrane, creating a proton gradient.
The electrons then proceed through a series of cytochrome complexes, each one accepting electrons from the previous complex and releasing energy to further pump protons across the membrane. These complexes work in tandem, akin to a relay team, efficiently transferring electrons and generating a significant proton gradient.
The final electron acceptor in the ETC is oxygen. As the electrons reach the terminal cytochrome complex, they combine with oxygen and protons to form water. This process, known as oxidative phosphorylation, releases a substantial amount of energy, which is harnessed to drive the synthesis of ATP molecules.
Oxidative Phosphorylation
- Explain the final stage of aerobic respiration, where ATP is synthesized using the proton gradient.
Oxidative Phosphorylation: The Powerhouse of Cells
In the grand orchestra of cellular respiration, oxidative phosphorylation takes the stage as the maestro, orchestrating the final symphony of energy production. It’s the culmination of a complex dance, where protons dance across the mitochondrial inner membrane, their movement fueling the creation of ATP, life’s essential energy currency.
The Proton Gradient: A Symphony of Energy
Oxidative phosphorylation begins with a proton gradient, a meticulously crafted imbalance of protons across the mitochondrial inner membrane. This gradient is the product of the electron transport chain, a series of protein complexes that pass electrons through redox reactions like stepping stones in a winding path. With each step, electrons lose energy, which is harnessed to pump protons from the mitochondrial matrix into the intermembrane space.
ATP Synthase: The Gatekeeper of Energy
At the heart of oxidative phosphorylation lies ATP synthase, a molecular gatekeeper that controls the flow of protons back into the matrix. As protons rush through ATP synthase’s labyrinthine structure, their downhill movement drives the synthesis of ATP from ADP and inorganic phosphate.
The Electron Transport Chain: A Conductor of Reactions
The electron transport chain, a series of four protein complexes, serves as the conductor of this symphony of reactions. Each complex passes electrons from one to another, releasing energy that fuels proton pumping. Complex I, the first in the chain, receives electrons from NADH and oxidizes them, transferring their energy to the proton gradient. Complex II similarly oxidizes FADH2, adding its energy to the protonic symphony.
Complex III and Complex IV continue the electron transfer, further increasing the proton gradient. Finally, Complex IV transfers electrons to oxygen, the ultimate electron acceptor, reducing it to water.
The Importance of Oxidative Phosphorylation
Oxidative phosphorylation is paramount to life on Earth. It provides the majority of energy used by cells, powering everything from muscle contractions to nerve impulses. Without this vital process, cellular respiration would falter, and life itself would cease to exist.
So, when you take a deep breath or perform an everyday task, remember the intricate dance of oxidative phosphorylation within your cells, the powerhouse that fuels your very existence.
Chemiosmosis: The Powerhouse of Cellular Respiration
Nestled within the mitochondria, the powerhouse of cells, lies a remarkable process known as chemiosmosis. It’s the final stage of cellular respiration, the dance that generates the energy that fuels life.
Imagine a proton gradient, a difference in the concentration of protons (H+) across the mitochondrial inner membrane. This gradient is like a tiny battery, storing potential energy. Chemiosmosis harnesses this energy to synthesize ATP, the universal energy currency of cells.
At the heart of chemiosmosis lies the electron transport chain, a series of protein complexes. As electrons pass through these complexes, their energy is used to pump protons across the inner membrane. This creates the proton gradient, with protons building up in the space between the membranes.
Like water rushing through a dam, protons flow back down the gradient through a channel called ATP synthase. As they pass through, they drive the rotation of a tiny rotor within ATP synthase. This spinning motion powers the synthesis of ATP.
ATP synthase is a remarkable molecular machine that couples the flow of protons to the synthesis of ATP. With each rotation, it grabs ADP (adenosine diphosphate) and inorganic phosphate (Pi) and joins them together to form ATP (adenosine triphosphate).
This process of chemiosmosis is essential for life. It generates the vast majority of the ATP that cells need to power their activities, from muscle contraction to nerve impulses to protein synthesis.
So, the next time you take a breath or lift a finger, remember the incredible power of chemiosmosis. It’s the silent symphony that fuels our every move and sustains the very essence of life.