Cellular Respiration: Unlocking Energy Production Through Electron Transport And Atp Synthesis
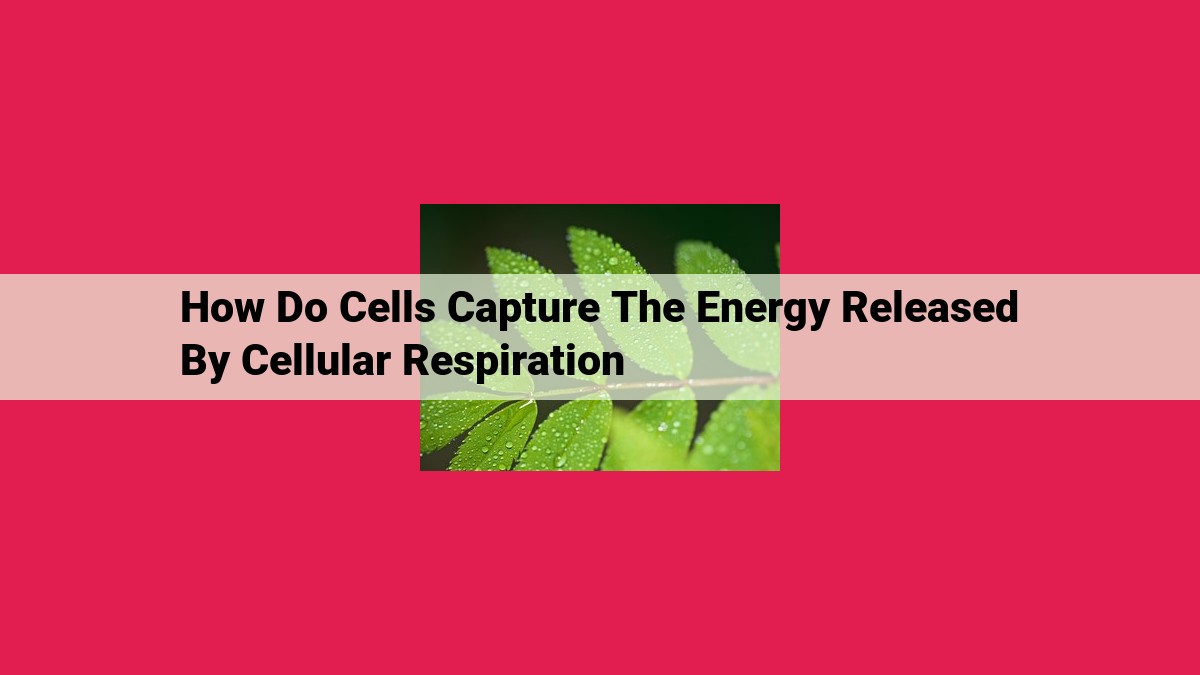
During cellular respiration, cells capture energy through a series of steps involving electron carriers (NADH and FADH2) and the electron transport chain. As electrons pass through the chain, protons are pumped across the mitochondrial membrane, creating a proton gradient. This gradient drives ATP synthesis via ATP synthase, an enzyme that harnesses the energy released by the proton flow to produce ATP, the primary energy currency of cells. This intricate process ensures that the energy released during glucose breakdown is efficiently converted into usable ATP, powering cellular processes and activities.
- Define cellular respiration and its significance in providing energy for cells.
Cellular Respiration: The Powerhouse of Cells
Imagine a bustling city, where tiny machines and pathways work tirelessly to generate the energy that fuels all life processes within cells. This intricate energy production system is known as cellular respiration, the lifeblood of your cells.
Cellular respiration is a series of chemical reactions that convert the energy stored in glucose, a type of sugar, into adenosine triphosphate (ATP), the primary energy currency of our bodies. ATP serves as the “fuel” for various cellular activities, such as muscle contraction, protein synthesis, and chemical transport.
The journey to energy production begins with the breakdown of glucose into smaller and more manageable molecules. This process occurs in the cytoplasm, the fluid-filled interior of the cell. The energy harvested during this breakdown process is captured by two electron carriers known as nicotinamide adenine dinucleotide (NADH) and flavin adenine dinucleotide (FADH2).
NADH and FADH2 are like messengers that carry electrons to the mitochondria, the powerhouses of the cell. Within the mitochondria, these electron carriers pass their electrons to a series of proteins known as the electron transport chain. This chain resembles a winding river, where the electrons flow down a “waterfall” of proteins, releasing energy at each step.
The energy released along this electron transport chain is harvested and stored as a proton gradient across the mitochondrial membrane. This concentration gradient creates an electrical potential, similar to a battery, driving the synthesis of ATP.
ATP synthase, a membrane-bound enzyme, acts like a turbine, allowing protons to flow down the gradient, spinning its gears and generating ATP. This process is akin to a waterwheel that converts the energy of flowing water into mechanical energy.
Cellular respiration is an awe-inspiring symphony of biological machinery, where glucose is transformed into ATP, powering the myriad of processes that sustain life. It’s a testament to the incredible complexity and sophistication of the living cell.
ATP: The Cellular Energy Currency
In the bustling metropolis of a living cell, energy is the lifeblood that powers every aspect of its existence. At the heart of this energy economy lies a remarkable molecule called adenosine triphosphate (ATP), the primary currency of cellular energy transactions.
Imagine ATP as a tiny battery, storing chemical energy within its three phosphate groups. Each phosphate bond, like a coiled spring, holds immense energy potential. When the cell requires a surge of power, these bonds are hydrolyzed, releasing energy that can be harnessed for various cellular activities.
This process of ATP hydrolysis is akin to snapping a rubber band. As the bond breaks, the energy is released, propelling the cellular machinery into action. This energy can be used to drive muscle contractions, fuel chemical reactions, or even power the blink of an eye.
Electron Carriers: The Unsung Heroes of Cellular Energy
In the intricate symphony of cellular respiration, where energy is extracted from glucose, there are two unsung heroes that play a crucial role in capturing this energy: NADH and FADH2. These electron carriers act as molecular messengers, transporting electrons that carry the potential for ATP production.
NADH: The Electron-Grabbing Powerhouse
Nicotinamide adenine dinucleotide (NADH) is the primary electron carrier in cellular respiration. As glucose is broken down, NADH grabs electrons from the breakdown products like a molecular vacuum cleaner. These electrons are tightly bound to NADH, ready to be released when needed.
FADH2: The Supporting Cast
Flavin adenine dinucleotide (FADH2) is another electron carrier, but it plays a supporting role to NADH. FADH2 captures electrons from a different set of breakdown products, providing an additional source of energy.
Transferring Electrons to the Electron Transport Chain
Once NADH and FADH2 have captured electrons, they pass them along to the electron transport chain, a series of protein complexes embedded in the mitochondrial membrane. This chain acts like an electrical wire, transferring electrons from one complex to the next.
As the electrons flow through the transport chain, they lose energy. This energy is used to pump protons across the mitochondrial membrane, creating a proton gradient. This gradient is the key to unlocking the final step of cellular respiration: ATP synthesis.
The Electron Transport Chain: Where Energy is Transported and Protons are Pumped
In the heart of our cells, a mesmerizing dance of energy transfer takes place. The electron transport chain, a remarkable molecular ensemble located within the mitochondrial membrane, plays a pivotal role in this energy ballet. It serves as a conduit for electrons, guiding them on a journey that ultimately leads to the production of ATP, the cellular energy currency.
As electrons embark on this adventure, they encounter a series of protein complexes, each meticulously designed to transfer electrons in a stepwise fashion. These complexes act like molecular pumps, pulsating protons across the mitochondrial membrane. With each proton that traverses the membrane, a tiny pocket of energy is created, akin to a dammed-up reservoir waiting to unleash its power.
The accumulation of these protonic reservoirs establishes a gradient, a disparity in concentration across the membrane. This gradient is the driving force behind the final act of cellular respiration: the synthesis of ATP.
Proton Gradient and ATP Synthesis
- Describe the creation of the proton gradient across the mitochondrial membrane.
- Explain the role of the proton gradient in driving ATP synthesis.
Proton Gradient and ATP Synthesis
As the electrons cascade down the electron transport chain, they release energy. This energy is harnessed to pump protons across the mitochondrial membrane, creating a proton gradient. This gradient is akin to a dammed-up reservoir, containing potential energy.
The proton gradient plays a crucial role in generating ATP, the cell’s primary energy currency. ATP synthase, an enzyme embedded in the mitochondrial membrane, acts as a molecular turbine. As protons flow down the gradient, they pass through ATP synthase, causing it to rotate.
This rotation triggers conformational changes within ATP synthase, enabling it to synthesize ATP from ADP (adenosine diphosphate) and inorganic phosphate. The energy stored in the proton gradient is thus converted into the chemical bonds of ATP.
The process of ATP synthesis is like a perpetual motion machine, driven by the constant flow of protons across the mitochondrial membrane. This energy-rich compound is then transported throughout the cell, where it fuels a multitude of cellular processes.
In summary, the proton gradient is the driving force behind ATP synthesis. As electrons flow down the electron transport chain, they pump protons across the mitochondrial membrane, creating a reservoir of potential energy. ATP synthase harnesses this energy to generate ATP, the universal energy currency of cells.
ATP Synthase: Harvesting Energy for ATP Production
- Describe the structure and function of ATP synthase as a membrane-bound enzyme.
- Explain the process of proton flow through ATP synthase and the conformational changes that lead to ATP synthesis.
ATP Synthase: Harvesting Energy for ATP Production
Cellular respiration, the powerhouse of cells, generates energy through a series of intricate steps. The electron transport chain pumps protons across the mitochondrial membrane, creating a proton gradient. This gradient is the driving force behind ATP synthesis, the final stage of cellular respiration that generates the body’s main energy currency: ATP.
At the heart of ATP synthesis lies ATP synthase, a membrane-bound enzyme complex. The key to its operation is proton flow. Protons from the gradient rush through a channel within ATP synthase, causing conformational changes that facilitate the production of ATP.
Imagine ATP synthase as a rotating door. As protons flow through the channel, the door spins. This spinning motion drives another component of the enzyme to undergo a series of shape changes. These shape changes ultimately lead to the synthesis of ATP from ADP and inorganic phosphate.
Essentially, the proton gradient acts like a hydroelectric dam, using the flow of protons to generate energy. ATP synthase harnesses this energy to power the synthesis of ATP, the cellular energy currency. Without this final step, the energy captured during cellular respiration would be lost.
ATP synthase is the culmination of cellular respiration, the process that powers our cells and bodies. It’s a testament to the elegance of biological systems, where the flow of protons through a membrane can be harnessed to produce the energy that sustains life.