Unlocking The Secrets Of Cell Structure And Function: A Comprehensive Guide
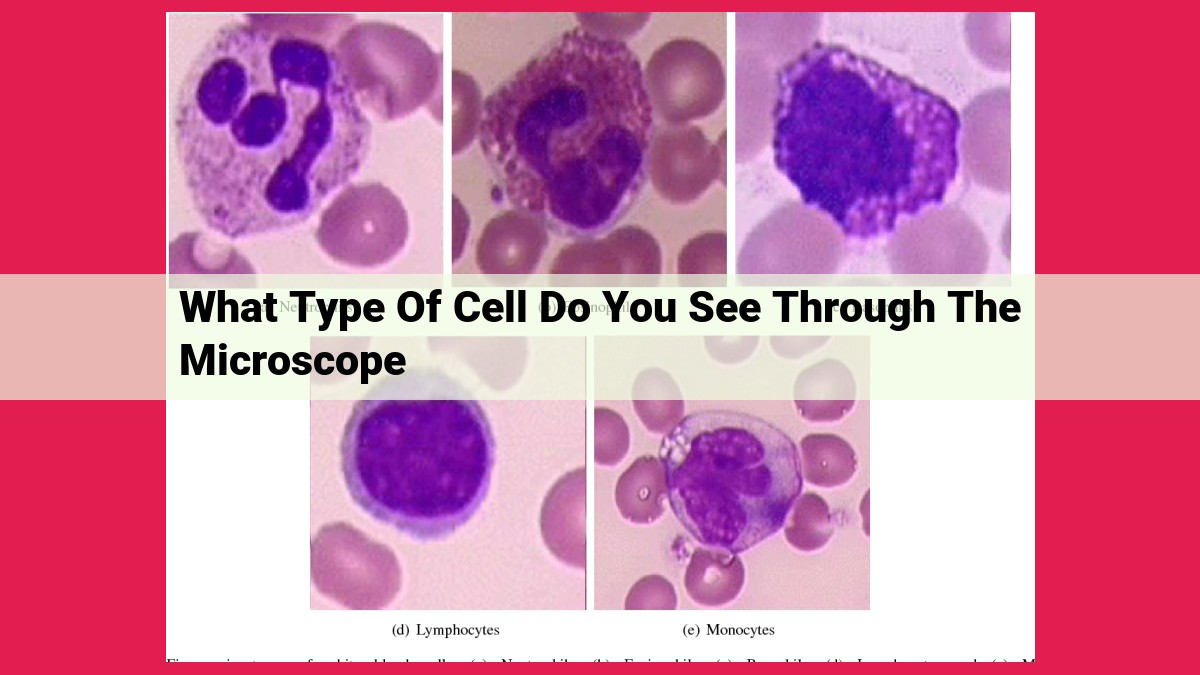
Through the microscope, we witness a myriad of cell types: from the simple prokaryotes to the complex eukaryotes, each with its distinct features and specializations. Plant cells boast cell walls and chloroplasts for photosynthesis, while animal cells lack these features. Differentiated cells contribute to organ function, with variations in size and shape corresponding to their specific roles. The cell wall or membrane protects and regulates permeability, while the nucleus houses the genetic material. Cytoplasm hosts organelles like mitochondria, Golgi apparatus, ribosomes, and endoplasmic reticulum, each performing vital cellular functions. These diverse cell types orchestrate a harmonious symphony of life, enabling organisms to thrive.
The Marvel of Cells: Unlocking the Secrets of Life’s Building Blocks
Imagine a world hidden within us, a realm where microscopic wonders dance and play, shaping the very essence of our being. This is the realm of cells, the fundamental units of life. From the simplest bacteria to the most complex organisms, cells are the architects of life’s tapestry, performing an astounding array of tasks that sustain our existence.
In this journey into the microscopic, we will uncover the secrets of these cellular marvels. We will explore their remarkable diversity, from the tiny prokaryotes to the intricate eukaryotes, and delve into the differences that define plant and animal cells. We will unravel the mysteries of specialized cells and witness the breathtaking adaptations that allow them to perform their unique functions.
As we navigate this cellular landscape, we will encounter the enigmatic cell wall and the permeable cell membrane, guardians of the cell’s integrity. We will uncover the secrets of the nucleus, the command center of the cell, and marvel at the cytoplasm, a bustling hub of cellular activity. We will decipher the functions of organelles, specialized structures dedicated to specific tasks, and marvel at the efficiency with which they orchestrate cellular life.
Join us on this captivating odyssey into the world of cells, where we will unlock the secrets of life’s building blocks and appreciate the intricate harmony that sustains our very existence.
The Divide: Unveiling the Two Cell Empires – Eukaryotic vs. Prokaryotic
Introduction
At the heart of every living organism, an enigmatic microscopic world thrives. Within this realm reside the fundamental units of life – cells. Among the vast diversity of cells, two empires reign supreme: the eukaryotic and the prokaryotic cells. Embark on a journey to unravel the captivating tale of their distinctions, deciphering the blueprints that shape their very existence.
Cell Size: A Tale of Giants and Dwarfs
Eukaryotic cells, the giants in this cellular realm, can stretch up to tens of micrometers in diameter, dwarfing their prokaryotic counterparts. Prokaryotes, on the other hand, are miniature marvels, typically hovering around a few micrometers in size. This disparity in size reflects the contrasting complexity and functions of these two cellular domains.
Structural Divide: Complexity Unfolds
The intricate architecture of eukaryotic cells stands in stark contrast to the simpler design of prokaryotic cells. Eukaryotes possess a membrane-bound nucleus, safeguarding their genetic material. This nuclear sanctuary houses the cell’s DNA, the blueprint for life. Prokaryotes, lacking this nuclear enclosure, have their DNA floating freely within the cell.
Organelle Odyssey: A Symphony of Specialization
Eukaryotic cells are teeming with specialized compartments known as organelles, each performing a specific cellular function. Mitochondria, the energy powerhouses, generate cellular fuel. Endoplasmic reticulum and Golgi apparatus, the cellular postal service, process and distribute proteins. Prokaryotes, while lacking these elaborate organelles, have simpler structures that perform similar tasks.
A Tapestry of Life: Diversity in Motion
The eukaryotic and prokaryotic cell empires encompass an astounding diversity of organisms. Eukaryotes dominate the complex world of multicellular plants, animals, and fungi. Prokaryotes, the pioneers of life, thrive in diverse environments, from scorching hot springs to the icy depths of the ocean. This remarkable range of habitats showcases the adaptability and resilience of these cellular titans.
Conclusion
The divide between eukaryotic and prokaryotic cells is a testament to the boundless diversity of life’s blueprints. The complexity of eukaryotes, with their membrane-bound nucleus and specialized organelles, enables multicellular complexity and biological wonders like ourselves. Prokaryotes, despite their simplicity, survive in a myriad of environments, proving that even in the smallest of cells, life’s ingenuity knows no bounds.
Plant vs. Animal Cells: A Tale of Two Kingdoms
In the vast realm of living organisms, two distinct kingdoms stand out: plants and animals. Their cells, the fundamental building blocks of life, share some essential features but also exhibit striking differences that reflect their unique adaptations and functions.
Cell Wall: The Plant’s Armor
One of the most prominent distinctions between plant and animal cells lies in the presence or absence of a cell wall. This rigid structure, found exclusively in plant cells, provides support, protection, and shape. Made of cellulose, a strong and fibrous material, the cell wall allows plants to withstand the forces of gravity and keep their tissues upright.
Chloroplasts: The Green Powerhouses
Another key difference is the presence of chloroplasts in plant cells. These organelles, often referred to as the “green powerhouses,” contain chlorophyll, a pigment that captures sunlight energy. This energy is then harnessed to convert carbon dioxide and water into glucose through the process of photosynthesis. As a result, plants are self-sufficient organisms that can synthesize their own food from inorganic compounds.
Vacuoles: Storage Giants
In contrast to plant cells, animal cells lack chloroplasts but possess prominent vacuoles. These fluid-filled sacs serve as storage compartments for various substances, including water, ions, waste products, and nutrients. In plant cells, however, vacuoles are even more extensive and usually occupy most of the cell’s volume. They provide turgidity, helping plants resist wilting and maintaining their shape.
Diversity in Form and Function
Beyond these fundamental differences, plant and animal cells exhibit great diversity in their shapes and sizes. Plant cells are typically rectangular or polygonal, whereas animal cells can vary widely in shape, depending on their function. For instance, muscle cells are long and spindle-shaped, while nerve cells have long, branching extensions. This diversity reflects the specialized roles that cells play within their respective organisms.
The intricate interplay of plant and animal cells is a testament to the wonders of biological diversity. Their unique characteristics, from the rigid cell wall and green chloroplasts of plants to the storage vacuoles and diverse shapes of animal cells, enable these organisms to thrive in their specific environments and perform their essential functions in the ecosystem.
Specialized Cells: Guardians of Tissue and Organ Function
In the intricate tapestry of life, cells play a pivotal role, orchestrating the symphony of life’s processes. Specialized cells, like master craftsmen, are tailored to specific tasks, ensuring the seamless functioning of tissues and organs. This remarkable process, known as cell differentiation, transforms unspecialized cells into highly sophisticated specialists.
As embryonic cells embark on their developmental journey, they encounter a cascade of signaling molecules that guide their destiny. These signals trigger changes in gene expression, leading to the production of specialized proteins that shape the cell’s structure and function. Through this intricate process, cells acquire unique characteristics, both in their appearance and their ability to perform specialized tasks.
Specialized cells are the building blocks of complex organisms, contributing to the diversity of tissues and organs we see in nature. Muscle cells, with their incredible contractile power, enable movement and locomotion. Nerve cells, with their intricate network of connections, transmit electrical signals that govern communication and thought. Epithelial cells, lining our body’s surfaces, protect from external threats and regulate the exchange of substances.
Each specialized cell type is exquisitely adapted to its specific role. Red blood cells, with their unique shape and lack of nucleus, efficiently transport oxygen throughout the body. White blood cells, guardians of our immune system, identify and eliminate pathogens that threaten our well-being. Stem cells, with their remarkable potential, hold the promise of regenerating damaged tissues and organs.
The specialization of cells is a testament to the incredible diversity and adaptability of life. Through this process, cells become highly efficient at performing their specialized tasks, ensuring the harmonious functioning of the entire organism. Truly, specialized cells are the unsung heroes of our bodies, tirelessly working behind the scenes to maintain our health and well-being.
**Cell Size and Shape: Adaptations for Diverse Roles**
In the vast microscopic world of cells, size and shape are not mere aesthetics but functional adaptations that enable cells to perform their specialized roles within the organism. From the tiniest bacteria to the sprawling nerve cells, cell dimensions and contours vary dramatically, each tailored to a particular function.
Take the red blood cell, a small, disk-shaped wonder. Its flattened, donut-like structure allows it to squeeze through narrow blood vessels, delivering oxygen to every nook and cranny of the body. Its small size ensures a large surface area-to-volume ratio, maximizing oxygen diffusion.
At the other end of the spectrum, nerve cells, also known as neurons, possess slender, elongated extensions called axons. These axons can stretch over vast distances, carrying electrical signals throughout the nervous system with incredible speed and efficiency. Their elongated shape facilitates rapid communication between distant parts of the body.
Even within the same tissue, cells may exhibit remarkable shape diversity. Consider muscle cells: their elongated, cylindrical shape allows them to contract and relax, providing the force for movement. In contrast, fat cells are round and bulge-shaped, optimized for energy storage.
Cell size and shape also play crucial roles in cellular processes. For example, small, spherical cells are more efficient at diffusion, while larger, irregular-shaped cells can accommodate more complex organelles and perform specialized functions.
The diversity of cell size and shape is a testament to the ingenuity of nature. Each adaptation has evolved to meet the specific demands of a cell’s role, ensuring the harmonious functioning of the entire organism.
Cell Wall vs. Cell Membrane: Guardians of Cell Integrity and Permeability
In the realm of cells, the cell wall and cell membrane stand as sentinels, safeguarding their precious contents and regulating the flow of substances that enter and exit. While both structures serve vital roles in cellular protection and permeability, they exhibit distinct characteristics that reflect the unique adaptations of different cell types.
The cell wall, a rigid exoskeleton exclusive to plant cells, provides structural support and protection against mechanical stress. Composed of cellulose, a sturdy polysaccharide, the cell wall maintains the cell’s shape and prevents it from bursting under the pressure of its internal fluids. It also acts as a barrier against pathogens and environmental toxins, shielding the cell from harm.
In contrast, the cell membrane, a flexible and semi-permeable barrier, is found in both plant and animal cells. Composed of a lipid bilayer, the cell membrane forms a fluid mosaic that allows essential substances to pass through while restricting the entry of harmful molecules. This selective permeability is crucial for maintaining cellular homeostasis and regulating the exchange of nutrients, waste products, and signaling molecules.
The cell wall and cell membrane differ significantly in their composition and permeability. The cell wall is a rigid structure made of cellulose, while the cell membrane is a flexible bilayer composed of lipids and proteins. This difference in composition reflects their respective functions: the cell wall provides structural support, while the cell membrane regulates permeability and allows for cellular processes.
The cell wall and cell membrane are essential components of cells, providing protection and regulating permeability. While they both serve these vital functions, their distinct structures and compositions reflect the diverse adaptations of different cell types. The cell wall, exclusive to plant cells, provides structural support, while the cell membrane, present in both plant and animal cells, regulates the flow of substances and maintains cellular homeostasis. Together, these structures ensure the integrity and functionality of cells, allowing them to thrive in their respective environments.
The Central Command: The Nucleus
The Nucleus: The Control Center of the Cell
Within the bustling city-state of the cell, there lies a central command, a nucleus, the control center that governs all cellular activities. Enclosed within a fortified nuclear membrane, this organelle is the nerve center of the cell, orchestrating essential functions that ensure the cell’s survival and proper operation.
The Genetic Powerhouse
The nucleus is the guardian of the cell’s genetic blueprint, the DNA. These long, coiled molecules reside within the nucleus, carrying the genetic instructions for every protein and cellular structure. The nucleus acts as a command center where gene expression is carefully regulated, controlling when and how genetic information is translated into the proteins that carry out cellular functions.
The Master of Cell Division
When the cell is ready to divide and create a new generation of cells, the nucleus takes center stage. It orchestrates the meticulous process of mitosis, where each chromosome is precisely duplicated and separated into two identical sets. This division ensures that each new cell receives a complete copy of the genetic material, essential for growth and reproduction.
A Well-Protected Fortress
The nucleus is not merely a genetic library but also a well-protected fortress. The nuclear membrane, a double-layered barrier, selectively controls the movement of molecules, preventing unauthorized access to the genetic material. This protective layer ensures that the integrity of the genetic code is maintained, safeguarding the cell’s identity and function.
The Cytoplasm: A Hub of Cellular Activity
The cytoplasm is the gelatin-like substance that fills the cell outside the nucleus. It is a busy hub of cellular activity, containing a vast network of organelles, metabolic processes, and the cytosol – a fluid-filled matrix. Just like a well-run city, the cytoplasm coordinates and facilitates a multitude of essential cellular functions.
Organelles: Specialized Structures, Specific Tasks
Within the cytoplasm reside various organelles, each with a unique and specialized role in cellular life. Mitochondria are the energy factories of the cell, generating ATP through cellular respiration. Ribosomes, those tiny protein builders, are responsible for protein synthesis. The endoplasmic reticulum is a maze of membranes where proteins are folded, modified, and transported. And the Golgi apparatus acts as the cellular post office, packaging and distributing proteins and other molecules to their designated destinations.
Metabolic Processes: Fueling Cellular Activities
The cytoplasm is also the site of various metabolic processes that sustain the cell. Glycolysis, the breakdown of glucose for energy, occurs in the cytoplasm. Lipid synthesis, the creation of fats and oils, takes place on the membranes of the endoplasmic reticulum. And protein degradation, the breaking down of proteins, happens in specialized organelles called lysosomes.
Cytosol: A Dynamic Matrix
The cytosol is the fluid-filled matrix that fills the cytoplasm. It is not simply an empty space but a dynamic network of proteins, ions, and molecules. The cytosol is involved in various cellular processes, such as signal transduction, protein synthesis, and waste removal. It is the cytoplasm’s fluid highway, transporting essential materials throughout the cell.
Without the cytoplasm and its myriad components, the cell would be a lifeless entity. The cytoplasm is the bustling metropolis of the cell, coordinating and supporting a symphony of cellular activities that keep the cell – and, ultimately, the organism – alive and functioning.
Organelles: The Specialized Machinery of Life
In the bustling metropolis of a cell, organelles serve as the specialized machinery that keeps the city running smoothly. These tiny compartments, each with its unique function, play a crucial role in ensuring the cell’s survival and proper operation.
Compartmentalization: A Tale of Order
Organelles are not haphazardly scattered within the cell. Instead, they are neatly compartmentalized, allowing for efficient and organized execution of cellular processes. This compartmentalization is essential to prevent interference between different functions and maintain cellular harmony.
Specialization: A Symphony of Expertise
Each organelle is a master of its craft, performing a specific task that contributes to the overall health and functioning of the cell. Whether it’s protein synthesis, energy production, or waste disposal, every organelle has a vital role to play.
Meet the Organelle Team:
- Mitochondria: The powerhouses of the cell, they generate energy through cellular respiration, fueling the cell’s activities.
- Ribosomes: The protein builders, they assemble amino acids into proteins, the building blocks of life.
- Endoplasmic Reticulum: The protein processor and transporter, it modifies proteins and transports them within the cell.
- Golgi Apparatus: The cellular post office, it packages and distributes proteins and lipids to their destinations.
- Lysosomes: The cellular cleaners, they digest waste and cellular debris, keeping the cell tidy.
The diverse array of organelles within a cell is a testament to the complexity and sophistication of life. Each organelle, with its specialized function, contributes to the harmonious symphony of cellular activity. From protein synthesis to waste disposal, organelles ensure the smooth functioning and well-being of the cell, ultimately contributing to the overall health of the organism.
Vacuoles: The Cellular Storerooms and Waste Managers
Within the bustling realm of cells, there exists a fascinating organelle known as the vacuole. These membrane-bound compartments serve as storage units, waste disposal facilities, and buoyancy regulators, playing a pivotal role in maintaining cellular homeostasis and supporting diverse life processes.
In plant cells, vacuoles are particularly prominent, often occupying a large portion of the cell’s volume. These spacious compartments act as reservoirs for water, aiding in cell turgidity and providing structural support. When water enters the vacuole, the cell expands, creating a rigid framework that enables the plant to stand upright.
Vacuoles also serve as storage havens for a variety of substances. They stockpile nutrients, such as sugars, amino acids, and minerals, which can be released as needed to fuel cellular processes. Additionally, plant vacuoles can store specialized compounds, such as pigments that give flowers their vibrant colors, and defensive substances that protect against pests and diseases.
Beyond their storage capabilities, vacuoles play a crucial role in waste management. They house waste products and degraded cellular components, effectively recycling these materials to reduce cellular toxicity. Some vacuoles contain digestive enzymes that break down waste matter, preparing it for disposal.
In some single-celled organisms, such as Paramecium, a specialized vacuole known as the contractile vacuole, functions as a pumping mechanism to expel excess water. This is essential for maintaining the cell’s water balance and preventing rupture.
In summary, vacuoles are versatile organelles that contribute significantly to cellular functioning. They store resources, eliminate waste, regulate buoyancy, and protect the cell from harmful substances. Their diverse roles underscore the intricate symphony of life within the microscopic realm.
Chloroplasts: The Photosynthetic Powerhouses
In the intricate world of cells, there exists a unique organelle that holds the key to life’s most fundamental process: photosynthesis. Chloroplasts, found exclusively in plant cells, are the miniature factories responsible for converting sunlight into chemical energy, providing sustenance to all living organisms.
The Green Guardians of Life
Imagine a microscopic solar panel nestled within a plant cell. That’s where chloroplasts reside, their verdant hue a testament to their vital role. These chlorophyll-packed organelles are the gatekeepers of photosynthesis, a process that harnesses light energy from the sun to transform carbon dioxide and water into glucose, the building block of life.
A Complex Symphony of Light
Within chloroplasts, a intricate dance of molecules takes place, a symphony of light conversion and energy storage. Special pigments, known as chlorophylls, capture sunlight and channel its energy into a series of electron transfers. These electrons are then used to reduce carbon dioxide into glucose, a sugar molecule that plants use as fuel.
The Byproduct of Life
As a byproduct of photosynthesis, chloroplasts release oxygen into the atmosphere, a vital breath for all aerobic organisms. This constant infusion of oxygen sustains life on Earth, making chloroplasts the cornerstone of our planet’s ecosystem.
Adapting to Diverse Environments
Just as plants have adapted to a myriad of environments, so too have chloroplasts. In plants that live in low-light conditions, chloroplasts may be larger and more numerous to maximize light absorption. Conversely, shade-loving plants may have fewer, smaller chloroplasts to minimize energy loss.
The Foundation of Life’s Web
Chloroplasts are not mere organelles; they are the pillars upon which life as we know it rests. Without their ability to capture sunlight and convert it into energy, the food chains that sustain our planet would collapse, and life as we know it would cease to exist.
Chloroplasts stand as a testament to the incredible diversity and complexity of life’s building blocks. As the photosynthetic powerhouses of the plant world, they play a pivotal role in the intricate web of life, providing sustenance and oxygen to all living organisms. Their ability to harness the energy of the sun is a marvel of nature, a reminder of the extraordinary interconnectedness of our planet’s ecosystem.
Mitochondria: The Powerhouses of Cells
Every cell within your body, from the tiniest bacterium to the complex neurons in your brain, contains its own energy-generating powerhouses known as mitochondria. These tiny organelles are essential for cellular respiration, the process by which cells convert nutrients into ATP, the universal currency of energy within living organisms.
ATP: The Fuel of Cellular Processes
ATP, or adenosine triphosphate, is the molecule that powers all cellular activities, from muscle contraction to nerve impulse transmission. Mitochondria are responsible for synthesizing ATP through a process called oxidative phosphorylation. This process involves the transfer of high-energy electrons along a series of proteins in the mitochondrial inner membrane, ultimately generating a proton gradient that drives the synthesis of ATP.
Double Membranes, Specialized Compartments
Mitochondria possess a unique double membrane structure. The outer mitochondrial membrane is relatively permeable, allowing small molecules to pass through freely. The inner mitochondrial membrane, however, is highly impermeable, creating a specialized compartment within the matrix. This compartment is where the enzymes involved in oxidative phosphorylation reside.
The Electron Transport Chain: A Symphony of Proteins
The inner mitochondrial membrane contains a series of protein complexes known as the electron transport chain. These complexes act as a conduit for electrons, passing them from one complex to the next. As electrons move through the chain, their energy is harnessed to pump protons across the membrane, creating the proton gradient necessary for ATP synthesis.
Oxidative Phosphorylation: Energy from Oxygen
The final step in cellular respiration is oxidative phosphorylation. In this process, protons flow back across the inner mitochondrial membrane through a protein complex called ATP synthase. This flow of protons drives the rotation of ATP synthase, which in turn synthesizes ATP from ADP (adenosine diphosphate). The ultimate electron acceptor in this process is oxygen, which combines with protons and electrons to form water.
Essential for Cellular Metabolism
Mitochondria are indispensable to cellular metabolism, the sum of all chemical processes within a cell. Without these energy powerhouses, cells would quickly run out of fuel, leading to cell death and ultimately organismal failure. The number and activity of mitochondria within a cell directly correlate with its energy demands. For example, muscle cells, which require large amounts of energy for contraction, contain a high density of mitochondria.
Mitochondria are the unsung heroes of cellular life, providing the energy that fuels all cellular processes. Their unique double membrane structure, specialized compartments, and electron transport chain enable them to efficiently harness the energy from nutrients and convert it into ATP. Without mitochondria, cells would cease to function, and life as we know it would not be possible.
Ribosomes: The Protein-Synthesizing Machines of Cells
In the bustling metropolis of a cell, ribosomes stand as the master builders, meticulously assembling the proteins that are the foundation of life’s processes. These tiny, complex structures are essential for every cell, from the humblest bacterium to the most complex organism. Ribosomes are the unsung heroes of the cellular world.
Protein Synthesis: A Ribosomal Symphony
Ribosomes are protein-synthesizing machines, responsible for transforming genetic instructions encoded in DNA into the functional proteins that orchestrate all cellular activities. This intricate process, known as protein synthesis or translation, involves the following steps:
- Initiation: Ribosomes recognize the start codon on an messenger RNA (mRNA) molecule and bind to it.
- Elongation: Ribosomes decode the mRNA sequence, one codon at a time, and match the corresponding amino acids from the cellular pool.
- Termination: Ribosomes reach a stop codon and release the newly synthesized protein chain.
Ribosomal Location: Inside and Out
Ribosomes can be found both freely floating in the cytoplasm and bound to the endoplasmic reticulum (ER), a network of membranes that plays a crucial role in protein processing. Ribosomes attached to the ER are called bound ribosomes, while those in the cytoplasm are known as free ribosomes.
Bound ribosomes primarily synthesize proteins that are destined to be secreted out of the cell, while free ribosomes produce proteins that function within the cytoplasm.
The Structure of a Ribosome
Ribosomes are composed of two subunits, a large subunit and a small subunit. Each subunit is made up of a complex of ribonucleic acid (rRNA) molecules and proteins. The large subunit contains the catalytic center where peptide bonds are formed, linking amino acids together to create proteins.
Ribosomes: Key Players in Cellular Processes
Ribosomes play a pivotal role in numerous cellular processes, including:
- Protein synthesis: Ribosomes are the gatekeepers of protein production, essential for cell growth, repair, and function.
- Cell differentiation: Ribosomes provide the molecular building blocks for specialized cells to develop and perform their unique roles.
- Metabolism: Ribosomes synthesize enzymes that catalyze chemical reactions, enabling cells to extract energy and perform metabolic processes.
The Endoplasmic Reticulum: Protein Processing and Transport
Imagine the endoplasmic reticulum (ER) as a bustling factory within the cell, where proteins and lipids are meticulously processed. This labyrinthine network of membranes stretches throughout the cytoplasm, performing a crucial role in the intricate dance of cellular life.
The ER is divided into two distinct regions:
- Rough Endoplasmic Reticulum (RER): Studded with ribosomes, these membranes are responsible for protein synthesis. As nascent proteins emerge from the ribosomes, they enter the RER lumen, where they undergo folding and other modifications.
- Smooth Endoplasmic Reticulum (SER): Lacking ribosomes, these membranes specialize in lipid synthesis and transport. They produce and store important lipids such as phospholipids and steroids.
The RER transports its protein cargo from the lumen to various destinations within and outside the cell. Proteins destined for secretion or incorporation into the plasma membrane are packaged into vesicles called Golgi vesicles by a specialized region of the RER known as the transitional ER.
The SER, on the other hand, is involved in detoxifying drugs and other xenobiotics, metabolizing carbohydrates, and regulating calcium levels within the cell. It also plays a role in the synthesis of hormones and other molecules involved in cellular signaling.
The endoplasmic reticulum is not just a passive pipeline for protein and lipid production, but an active participant in cellular homeostasis and stress responses. When cells are under stress, the ER can trigger a signaling pathway known as the unfolded protein response (UPR), which halts protein synthesis and activates processes to alleviate the stress.
In conclusion, the endoplasmic reticulum is a vital organelle that orchestrates the production, processing, and transport of proteins and lipids. Its intricate structure and diverse functions are essential for the overall health and well-being of the cell.
The Golgi Apparatus: The Cellular Post Office
In the bustling metropolis of a cell, where life’s essential processes unfold, there exists a remarkable organelle known as the Golgi apparatus. Like a cellular post office, it plays a pivotal role in processing, packaging, and distributing diverse molecules throughout the cell.
This intricate organelle consists of a series of flattened membranes called cisternae, stacked one upon another. As proteins and lipids synthesized by the cell’s ribosomes and endoplasmic reticulum (ER) enter the Golgi, they undergo a series of meticulous modifications.
Enzymes within the Golgi apparatus add, remove, or rearrange sugar molecules, creating a variety of glycans that adorn proteins and lipids. These glycans not only enhance protein stability but also serve as molecular tags, guiding them to their designated destinations.
Once modified, the proteins and lipids are packaged into membrane-bound vesicles. These vesicles then bud off from the Golgi, carrying their precious cargo to various parts of the cell. Some vesicles fuse with the plasma membrane, releasing their contents outside the cell. Others target intracellular compartments, delivering essential materials for cellular maintenance and function.
The Golgi apparatus also acts as a quality control agent, ensuring that only properly folded and modified proteins are released. Defective proteins are marked for degradation and sent to lysosomes for disposal.
Without the Golgi apparatus, the cell would be a disorganized chaos. The essential molecules produced by the cell’s ribosomes and ER would remain unprocessed and inaccessible, crippling cellular functions. Like a well-run post office, the Golgi apparatus ensures the smooth and efficient delivery of vital cellular components, maintaining the delicate balance of life within the cell.
Lysosomes: The Cellular Cleaners
In the bustling city of a cell, there are many specialized departments working together to maintain order and function. Among these departments, lysosomes stand out as the dedicated cleaners, responsible for waste disposal and recycling.
Imagine lysosomes as tiny garbage trucks equipped with powerful digestive enzymes. These enzymes can break down a wide range of materials, including worn-out organelles, bacteria that have invaded the cell, and even damaged or misfolded proteins. This process of digestion is essential for the cell’s health, as it helps to clear away debris and make room for new and functional components.
Lysosomes are surrounded by a protective membrane that keeps their digestive enzymes from leaking out and damaging the rest of the cell. This membrane also allows lysosomes to fuse with other organelles, such as damaged mitochondria or peroxisomes, and use their enzymes to break them down.
In addition to waste disposal, lysosomes also play a role in cellular recycling. They contain enzymes that can break down complex molecules into simpler ones, which can then be reused by the cell. This process is crucial for maintaining the cell’s energy levels and ensuring that essential molecules are available for vital processes.
Lysosomes are essential for maintaining the health and functionality of the cell. Without them, waste would accumulate, damaged organelles would not be removed, and the cell would eventually become overwhelmed and die. Therefore, lysosomes are key players in the cell’s defense system, helping to keep it clean and running smoothly.