Factors Impacting Cell Size: Key Determinants For Cellular Function And Organismal Physiology
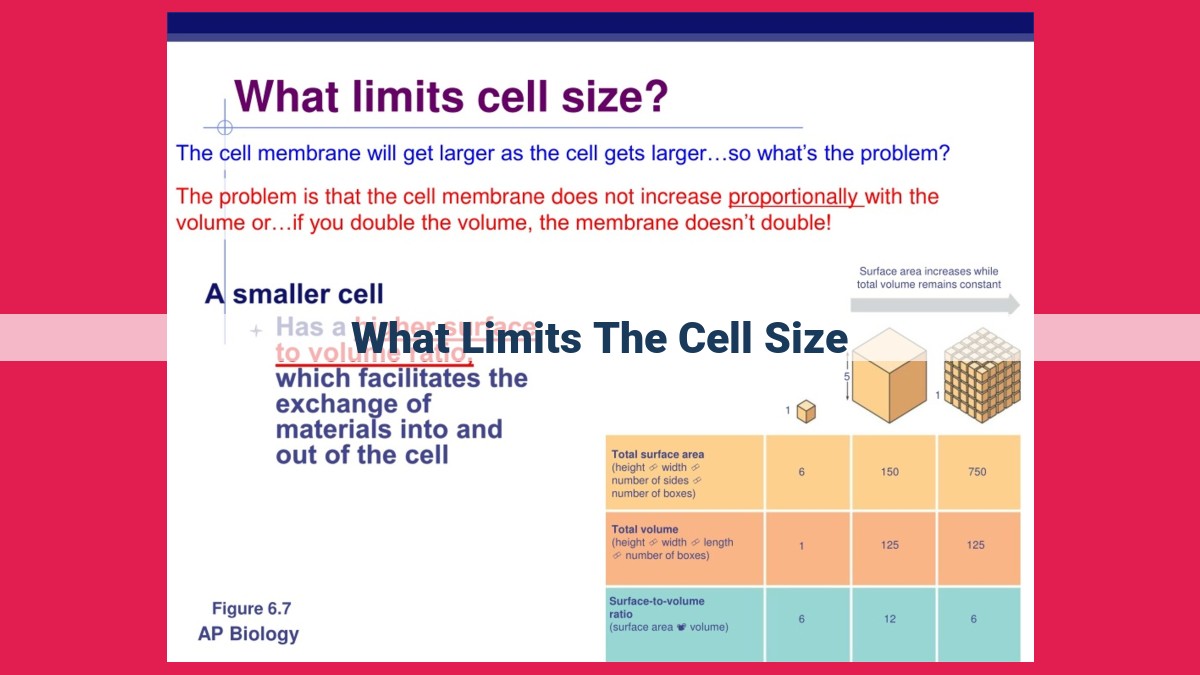
Cell size is crucial for cellular functions and organismal physiology. Factors limiting cell size include the surface area-to-volume ratio, nutrient delivery, waste removal, organelle size and number, genetic constraints, and physical forces. The surface area-to-volume ratio limits nutrient and oxygen exchange, while waste removal requires efficient transport mechanisms. Organelle size and number are constrained by cellular space and metabolic activity. Genetic factors regulate cell size, and physical forces influence cell shape and behavior. These limitations shape cellular functions, organismal physiology, and the evolution of cell size in different organisms.
The Intriguing Enigma of Cell Size: A Journey into the Microscopic World
Cells, the fundamental building blocks of all living organisms, exist in a vast spectrum of sizes, from the microscopic realm to the depths of our macroscale world. The size of a cell plays a crucial role in determining its functions and has profound implications for the physiology of multicellular organisms.
Just as the size of a ship governs its capacity and maneuverability, cell size influences cellular processes such as nutrient uptake, waste removal, and intracellular transport. The scaling of cells poses significant challenges, as cells must maintain a delicate balance between their surface area and volume.
As cells grow larger, their surface area increases less rapidly than their volume. This imbalance can limit the exchange of nutrients and waste products across the cell membrane. Diffusion, the passive movement of molecules, becomes less efficient as distance increases within the cell, imposing constraints on cell size.
Surface Area-to-Volume Ratio: Constraints on Cell Size
Imagine a town with no roads or highways. As the town grows, so does the challenge of moving people and goods around. Similarly, in cells, the surface area-to-volume ratio poses a fundamental constraint on cell size, as diffusion and metabolism become limiting factors.
Scaling and Cell Size:
As cells grow larger, their volume increases at a much faster rate than their surface area. This is because the surface area is a two-dimensional property, while the volume is three-dimensional. This scaling relationship has profound implications for cell function.
Diffusion and Metabolism:
Most cells rely on diffusion for the exchange of nutrients and waste products. As cells get larger, the distance between the cell’s nucleus and its outermost points increases. This longer distance slows down diffusion, making it more difficult to supply the cell’s interior with the necessary materials.
Moreover, metabolism, the process by which cells convert nutrients into energy, depends on chemical reactions that take place on the surface of organelles. As cell size increases, the ratio of surface area to volume decreases, reducing the number of organelles available for these reactions and limiting the cell’s ability to generate energy.
The surface area-to-volume ratio is a critical factor that influences cell size. As cells grow larger, they face challenges in diffusion and metabolism, which limit their ability to function effectively. This relationship has significant implications for cellular physiology and the evolution of cell size.
Nutrient Delivery: The Lifeline of Cellular Life
Cells, the fundamental units of life, rely on a continuous supply of nutrients to fuel their metabolic activities. The size of a cell, however, poses a significant challenge to this crucial delivery process.
As cells grow larger, their surface area-to-volume ratio decreases. This means that the surface area available for nutrient uptake becomes relatively smaller compared to the volume that needs to be sustained. Imagine a giant water balloon trying to absorb water through a small straw – it would be a slow and inefficient process.
To overcome this limitation, transport mechanisms have evolved to facilitate the efficient delivery of nutrients into cells. These mechanisms include passive diffusion, active transport, and facilitated diffusion.
In passive diffusion, molecules move from areas of high concentration to areas of low concentration without the need for energy input. This process is relatively slow but sufficient for small molecules like oxygen and carbon dioxide.
Active transport, on the other hand, uses energy to pump molecules against their concentration gradient. This mechanism is essential for transporting larger molecules like glucose and amino acids into cells.
Facilitated diffusion involves the use of carrier proteins embedded in the cell membrane to transport molecules. This process is faster and more specific than passive diffusion, allowing for the selective uptake of nutrients.
In larger multicellular organisms, specialized systems have evolved to enhance nutrient delivery. The circulatory system, composed of blood vessels, heart, and blood, transports nutrients throughout the body. The respiratory system, made up of lungs and airways, facilitates the uptake of oxygen from the environment. These systems work together to ensure a steady supply of nutrients to all cells in the organism.
The nutrient delivery process is a critical balancing act for cells. Cells must be large enough to accommodate their metabolic needs but small enough to efficiently absorb nutrients. The intricate interplay between cell size, transport mechanisms, and circulatory and respiratory systems ensures that cells receive the nourishment they need to thrive.
Waste Removal and Cell Size Limitations
The Crucial Role of Excretion
Just like us, cells need to get rid of their daily waste products to maintain a healthy and functional environment. This process, known as excretion, is essential for all cells, but it becomes even more critical in larger cells. Excess waste products can lead to cell malfunction, and in extreme cases, cell death.
Mechanisms of Waste Disposal
Cells employ several mechanisms to deal with waste removal. One common way is storage in specialized compartments called lysosomes. These cellular garbage bins contain enzymes that break down waste into smaller, less harmful substances. Alternatively, some waste products are transported directly to the cell membrane for elimination. This direct disposal is particularly important in cells with high metabolic activity, where waste is produced at a rapid pace.
Detoxification: A Vital Process
Certain waste products, such as ammonia, are toxic to cells. To protect themselves, cells have evolved sophisticated detoxification systems. These systems convert toxic waste into less harmful forms that can be safely excreted. One of the most crucial detoxification processes is the urea cycle, which converts ammonia into urea, a less toxic substance.
Implications for Cell Size
The need for effective waste removal poses a significant constraint on cell size. Larger cells have a greater surface area, which allows for more waste to enter. At the same time, the volume of the cell increases faster than its surface area, making it more difficult to remove waste efficiently. As a result, there is a limit to how large a cell can grow while maintaining proper waste management.
Example: Kidney’s Role in Large Organisms
In larger multicellular organisms, specialized organs such as the kidneys have evolved to handle the waste removal challenges. Kidneys act as miniature filtration systems, removing waste products from the bloodstream and producing urine. This efficient waste removal system allows cells in large organisms to grow beyond the size limits imposed by their own waste management capabilities.
Organelle Size and Number: The Balancing Act Within Cells
Cells, the fundamental building blocks of life, come in a remarkable range of sizes and shapes. While some cells, like bacteria, are microscopic, others, like neurons, can stretch over a meter in length. But what determines the size of a cell? And how do cells accommodate the vast array of organelles and molecules they need to function?
One factor that limits cell size is the availability of space. As a cell grows, the space it occupies increases at a rate proportional to the cube of its linear dimensions. However, the surface area of the cell membrane, through which nutrients enter and waste products leave, increases at a rate proportional only to the square of its linear dimensions. This means that as a cell gets bigger, its surface area-to-volume ratio decreases. This ratio is crucial because it affects how efficiently the cell can exchange materials with its surroundings.
To compensate for the decreasing surface area-to-volume ratio, cells have evolved a variety of strategies. One strategy is to increase the number of organelles within the cell. Organelles are membrane-bound compartments that perform specific functions within the cell. By increasing the number of organelles, cells can increase their surface area and thus improve their ability to exchange materials.
Another strategy is to compartmentalize the cell. Compartmentalization is the process of dividing the cell into smaller, specialized compartments. Each compartment can then perform a specific function, and the cell can avoid the problems associated with having a large, undivided space.
Finally, the size and number of organelles within a cell is also related to its metabolic activity. Cells that are more metabolically active require more organelles to perform the necessary chemical reactions. For example, muscle cells, which are highly active, have many mitochondria to produce the energy they need for contraction.
In conclusion, the size and number of organelles within a cell is a complex and finely tuned balance. Cells must carefully regulate the size and number of their organelles to ensure that they can function efficiently. The implications of these limitations for cellular functions and organismal physiology are vast, ranging from the development of diseases to the evolution of new species.
Genetic Constraints on Cell Size and Shape
At the heart of every cell lies its genetic code, the blueprint that governs its development and function. This code not only determines the cell’s identity but also plays a pivotal role in regulating its size and shape.
Gene Regulation
Genes, the fundamental units of heredity, contain instructions that guide the production of proteins, the building blocks of cells. These proteins, in turn, orchestrate a vast array of cellular processes, including those that determine cell size. The expression of genes, the process by which these instructions are translated into functional proteins, is tightly controlled by a complex network of regulatory factors. This dynamic regulation allows cells to fine-tune their size and shape in response to changing conditions within the body.
Evolutionary History
The genetic determinants of cell size have been shaped by millions of years of evolutionary history. Over time, natural selection has favored cells that possess optimal size for their specific ecological niche. For example, tiny bacteria thrive in environments with limited resources, while larger cells are better equipped to handle complex tasks in multicellular organisms. The evolutionary history of cell size limitations is thus a testament to the incredible power of genetic adaptation.
Implications for Cellular Functions and Organismal Physiology
The intricate interplay between genetic regulation and cell size has profound implications for cellular functions and organismal physiology. Smaller cells, with their increased surface-to-volume ratio, are more efficient at exchanging nutrients and waste products with their surroundings. This makes them ideal for specialized tasks such as nutrient absorption or cellular communication. Larger cells, on the other hand, can accommodate more complex internal structures, such as organelles and cytoskeletal elements, which enable them to perform more specialized functions.
By understanding the genetic constraints on cell size and shape, we gain a deeper appreciation for the interconnectedness of genetics, cellular biology, and the overall functioning of organisms. This knowledge不僅僅drives basic research but also has applications in fields such as medicine and biotechnology.
Physical Forces: Shaping the Cell’s Dimensions
Beyond the inherent constraints posed by surface area-to-volume ratio, nutrient delivery, waste removal, and genetic factors, physical forces play a significant role in shaping cell size and form. These external factors exert their influence through biomechanics, hydrodynamics, and gravity.
Biomechanics, the study of mechanical forces within living organisms, governs the cell’s ability to withstand deformation and maintain its structural integrity. Hydrodynamics, the study of fluids in motion, influences how fluids and solutes flow in and around cells, affecting nutrient and waste exchange. Gravity, the Earth’s gravitational pull, acts upon cells, influencing their orientation, shape, and migratory behavior.
The impact of these forces can be profound. For instance, cells in the circulatory system experience shear stress as they flow through blood vessels. This stress can influence their shape, deformability, and signaling responses. Conversely, cells in regions of the body subjected to compression must resist such forces to maintain their form and function.
Moreover, hydrodynamic forces play a crucial role in cell communication. By altering the flow of fluids around cells, neighboring cells can exchange signals and coordinate their activities. This is particularly important in multicellular organisms, where intricate networks of cells must work in harmony to maintain homeostasis.
In summary, physical forces impose additional constraints on cell size, influencing their shape, behavior, and function. These forces shape the way cells interact with their environment, contributing to the overall physiological functions of tissues, organs, and ultimately the entire organism.