Cell Membranes: Unlocking The Protective Barrier Of Cells For Optimal Seo
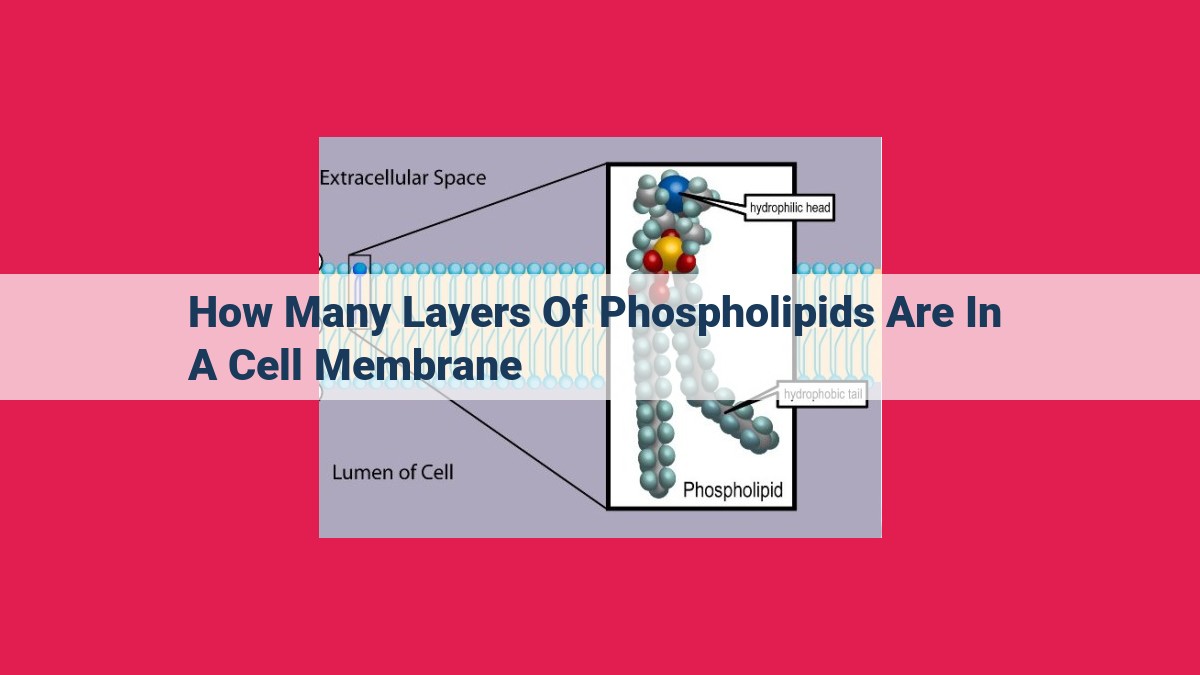
Cell membranes, defining the boundaries of cells, consist of a phospholipid bilayer, a two-layer structure. This bilayer comprises hydrophilic (water-loving) “heads” that face outward and inward and hydrophobic (water-fearing) “tails” that face inward, creating a barrier protecting the cell’s contents. The asymmetry between the outer and inner leaflets of the membrane, with distinct lipid and protein compositions, contributes to cellular function.
The Enigmatic Guardians of Life: A Journey into the Realm of Cell Membranes
In the bustling metropolis of a living cell, there exists a mysterious barrier that safeguards its precious contents and governs its interactions with the outside world. This remarkable structure is known as the cell membrane.
Imagine a thin, flexible sheet, composed of countless tiny molecules called phospholipids. These molecules are like microscopic building blocks, each with a water-loving head and a water-hating tail. When they come together, these phospholipids form a double layer, with their heads facing outward and their tails facing inward.
This phospholipid bilayer is the backbone of the cell membrane. It creates an impermeable barrier that protects the cell from harmful substances and maintains its internal environment. The cell membrane is not just a passive defense system, though. It’s a dynamic, living entity that regulates the flow of materials in and out of the cell.
Membrane Asymmetry: A Tale of Two Leaflets
The cell membrane is not a uniform structure. It has an inner leaflet and an outer leaflet. These leaflets differ in their composition, reflecting the cell’s specific needs. The inner leaflet is rich in phospholipids that contain a negatively charged head group. The outer leaflet is adorned with carbohydrates that are attached to proteins and lipids. This asymmetry is crucial for cell function, as it allows the cell to interact with its environment in a specific way.
Membrane Fluidity: The Dance of Molecules
The cell membrane is not a rigid structure. It is fluid, allowing its components to move and rearrange themselves. This fluidity is essential for cellular processes such as cell division and the transport of molecules across the membrane.
Membrane Potential: Sparks of Communication
The cell membrane also generates an electrical potential, known as the membrane potential. This potential is created by the asymmetric distribution of ions across the membrane. The membrane potential changes during action potentials, allowing cells to communicate with each other.
The Cell Membrane: A Protective Barrier with Remarkable Properties
In the bustling city of our body, our cells are like tiny fortresses, each guarded by a remarkable structure known as the cell membrane. This dynamic barrier not only separates the cell from its surroundings but also plays a crucial role in regulating what enters and exits the cell.
The foundation of the cell membrane lies in its unique composition, specifically the phospholipids that form its backbone. These phospholipid molecules are shaped like tiny balls, with a water-loving (hydrophilic) head and two water-hating (hydrophobic) tails.
As these phospholipids come together, they form a double layer, with their hydrophobic tails facing inward and their hydrophilic heads facing outward. This arrangement creates an efficient barrier that protects the cell from the watery environment outside. Imagine a line of umbrellas facing outward, shielding the cell from the rain.
The hydrophobic core of the cell membrane acts like an impenetrable wall, blocking the passage of unwanted substances. This barrier protects the cell’s delicate interior from harmful molecules while allowing essential nutrients to pass through.
The cell membrane is not a static structure but rather a dynamic entity, constantly adapting to its environment. Its fluidity allows the membrane to change shape, bend, and fuse with other membranes, enabling essential cellular processes such as cell division and the movement of molecules across the membrane.
Membrane Asymmetry: A Tale of Two Leaflets
Imagine your cell membrane as a double-layered door, with two distinct sides: the outer leaflet and the inner leaflet. While they may seem like mirror images, these leaflets are anything but equal.
Just like you have different clothes for different occasions, each leaflet has its own unique composition of phospholipids, proteins, and carbohydrates. The outer leaflet faces the outside world and is studded with molecules that interact with other cells, such as receptors and adhesion proteins. These proteins act as gatekeepers, determining what can enter and leave the cell.
On the other hand, the inner leaflet faces the inside of the cell and is home to molecules that help maintain the cell’s shape and connect to the cytoskeleton. It also contains pumps and channels that regulate the flow of molecules and ions across the membrane.
This asymmetry is crucial for cell function. It enables the cell to maintain different environments on either side of the membrane, which is essential for processes like cell signaling, nutrient uptake, and waste removal. Without this asymmetry, cells would struggle to survive and function properly.
Membrane Fluidity: The Dance of Cellular Life
Imagine a cell membrane as a bustling dance floor, with molecules of all shapes and sizes swirling and gliding effortlessly. This fluidity is essential for life, enabling a myriad of crucial cellular processes.
Cell division requires the fluid membrane to stretch and mold, allowing chromosomes to align and divide. Without this flexibility, the cell would be unable to replicate and pass on genetic material.
Molecular transport, the lifeblood of the cell, relies on membranes to shuttle molecules in and out. Small molecules slip through tiny channels, while larger molecules are carried by proteins that travel laterally within the membrane. This fluid movement ensures that cells can acquire nutrients, eliminate waste, and maintain their chemical balance.
Membrane fluidity also affects membrane permeability, controlling what substances can enter or exit the cell. The fluidity allows lipid molecules to move around, adjusting the size and shape of membrane pores. This dynamic regulation enables cells to fine-tune their interactions with the surrounding environment.
Factors such as temperature, lipid composition, and the presence of membrane proteins all influence membrane fluidity. Changes in these factors can alter the cell’s ability to perform various functions, impacting its overall health and well-being.
Membrane Potential: The Electrical Spark of Cell Communication
Cell membranes are not mere barriers, but rather dynamic gatekeepers that control the movement of ions and molecules across the cell. One of the most fascinating aspects of cell membranes is their electrical potential, known as membrane potential. This potential, like a tiny battery, plays a crucial role in regulating a cell’s communication with the outside world and enabling lightning-fast reactions.
Resting Potential: The Calm Before the Storm
Every cell maintains a resting potential, which is a stable electrical voltage difference across its membrane. This potential is due to the unequal distribution of ions (electrically charged particles) on either side of the membrane. Positively charged ions (cations) tend to accumulate outside the cell, while negatively charged ions (anions) are more abundant inside. This imbalance creates a voltage difference, much like a battery.
Action Potential: The Electrical Fireworks
When a cell receives a signal, such as a nerve impulse, the membrane potential undergoes a dramatic transformation. This process is called an action potential. During an action potential, voltage-gated ion channels in the membrane open, allowing sodium ions (Na+) to rush into the cell while potassium ions (K+) flow out. This influx of positive ions causes the inside of the cell to become more positive than the outside, creating a spike in voltage.
Wave of Excitation: Communicating the Message
The action potential does not stay put. It travels down the membrane as a wave of electrical excitation. This wave is caused by the opening of voltage-gated ion channels in neighboring regions of the membrane, which allows the flow of ions to continue. This propagation of the action potential enables cells to send rapid-fire signals to distant parts of the body.
Importance of Membrane Potential
Membrane potential is critical for many cellular functions, including:
- Cell-to-cell communication: Action potentials allow cells to transmit electrical signals to each other, facilitating communication within tissues and organs.
- Excitation of muscle and nerve cells: Action potentials trigger muscle contraction and nerve impulse transmission by causing calcium ions to enter the cell.
- Regulation of cellular metabolism: The membrane potential influences the activity of enzymes and other proteins involved in cellular metabolism.
In conclusion, the membrane potential is not just a passive electrical property of cells, but rather a dynamic force that governs cell communication and function. It is the electrical spark that enables cells to talk to each other and to respond to stimuli in a highly coordinated manner. Understanding membrane potential is essential for comprehending the intricate workings of life.
Membrane Proteins: Gatekeepers of the Cell’s Functions
Cell membranes are vital barriers that protect our cells from the environment. They also play a crucial role in cellular processes like transport, signaling, and adhesion. Embedded in these intricate membranes are membrane proteins, the gatekeepers of our cells’ functionalities.
Membrane proteins are classified based on their functions:
-
Transport proteins facilitate the movement of molecules across the membrane, acting as channels, pumps, or carriers. Integral to cellular functions, they regulate the balance of substances entering and leaving the cell.
-
Signaling proteins enable communication between the cell and its external environment. They bind to specific molecules and trigger a cascade of intracellular events, controlling cell growth, division, and differentiation.
-
Adhesion proteins link cells to one another and to the extracellular matrix. They provide structural support, facilitate cell-cell interactions, and contribute to tissue organization.
The diverse functions of membrane proteins are essential for cellular processes and overall organismal health. Their specific configurations and orientations within the membrane contribute to their unique roles in maintaining cellular homeostasis and mediating interactions with the external environment.
Membrane Carbohydrates: The Sugar Coating of Cells
Imagine your cells as tiny fortresses, surrounded by a protective moat—the cell membrane. But just as a moat has drawbridges and gates, the cell membrane has its own unique sugars that play a crucial role in cell recognition and adhesion.
These sugars, known as membrane carbohydrates, are like tiny beacons, helping cells to identify and interact with each other. They can be attached to proteins, forming glycoproteins, or to lipids, forming glycolipids. These sugar-studded molecules line the outer surface of the cell membrane, creating a “sugar coating” that influences how cells interact with their surroundings.
One of the most important functions of membrane carbohydrates is to differentiate between cells. Different cells have different sugar patterns, like unique fingerprints. These sugar fingerprints allow immune cells to distinguish between friendly and foreign cells and help guide cell migration during development and wound healing.
Membrane carbohydrates also play a key role in cell adhesion, the process by which cells stick to each other or to surfaces. These interactions are crucial for tissue formation and function. For example, carbohydrates on the surface of red blood cells help them adhere to the lining of blood vessels, ensuring proper blood flow.
In addition to cell recognition and adhesion, membrane carbohydrates play a role in immune responses, cell growth, and even cancer development. By understanding these sugar coatings, scientists can gain insights into various diseases and develop new therapies that target specific sugar molecules.
So, the next time you think of cell membranes, don’t just picture a bland barrier. Imagine a vibrant sugar-coated fortress, teeming with life and helping cells navigate their complex environment.
Membrane Transporters: Regulating Molecular Traffic Across Cell Membranes
Picture this: your cell membrane, the boundary between your cell and the bustling world outside. It’s not a static wall but a dynamic gateway, selectively allowing molecules to enter and exit. The gatekeepers of this complex transport system are membrane transporters.
Gateways of the Cell
Membrane transporters are specialized protein channels embedded in the cell membrane. They function like molecular locks and keys, recognizing specific molecules and facilitating their passage across the lipid barrier. This selective permeability allows the cell to maintain its internal environment and respond to external cues.
Types of Membrane Transporters
Membrane transporters come in various types, each with its unique mechanism:
- Passive transporters harness the energy gradient to facilitate the movement of molecules down their concentration gradients. Channel proteins form open pores, allowing the passage of specific ions, while carrier proteins bind to molecules and shuttle them across the membrane.
- Active transporters use energy from ATP to pump molecules against their concentration gradients. This energy-consuming process is crucial for maintaining essential cellular functions like ion balance and nutrient uptake.
- Facilitated diffusion transporters assist in the transport of molecules that cannot passively cross the membrane. They bind to molecules and facilitate their diffusion across the membrane.
Significance of Membrane Transporters
Membrane transporters are indispensable for:
- Nutrient uptake: Acquiring essential nutrients from the extracellular environment for cellular growth and metabolism.
- Ion balance: Maintaining proper ion concentrations within the cell, crucial for nerve impulse transmission, muscle contraction, and other cellular processes.
- Waste removal: Expelling metabolic waste products from the cell to maintain cellular homeostasis.
- Signal transduction: Mediating the influx of signaling molecules into the cell, triggering cellular responses to hormones and other external cues.
Membrane transporters are the gatekeepers of our cells, regulating the flow of molecules that shape our cellular functions. By understanding these molecular gatekeepers, we gain insights into the intricate workings of our bodies and the molecular mechanisms underlying health and disease.
Membrane Receptors: Explain how membrane receptors bind to ligands and trigger signal transduction pathways to alter cellular activity.
Membrane Receptors: The Gatekeepers of Cellular Communication
Within the bustling metropolis of the cell membrane, integral membrane proteins known as membrane receptors act as vigilant gatekeepers, orchestrating a delicate dance of communication between the inner sanctum of the cell and the dynamic world outside. These intricate receptors are the orchestrators of signal transduction, a vital process that converts external signals into tangible changes within the cell.
When a specific ligand, a molecule that binds to a receptor like a key fits into a lock, encounters its cognate membrane receptor, a captivating dance ensues. Binding of the ligand triggers a conformational change in the receptor, revealing a hidden dance floor where cellular events unfold.
Inside the cell, this dance becomes a cascade of intracellular messengers, known as second messengers, passing from one another like batons in a relay race. With lightning speed, second messengers rush to switch on protein kinases, enzymes that renovate proteins with molecular switches, altering their behavior and activating downstream processes.
From gene expression to cellular metabolism, signal transduction orchestrates a symphony of events that govern how our cells respond to their environment. Membrane receptors are the vigilant gatekeepers, standing guard at the cell’s boundaries, allowing only the most pertinent messengers to enter and shape the cell’s destiny.
Signal Transduction: The Cellular Communication Pathway
The final and equally fascinating chapter in our exploration of cell membranes delves into the realm of signal transduction, the intricate process that allows cells to communicate with each other and respond to their environment.
Imagine a bustling city where each cell is a resident, constantly receiving and sending messages. Signals, like messengers, arrive at the cell’s surface, where they bind to specific membrane receptors. These receptors, acting as gatekeepers, relay the signal into the cell.
Once inside, the signal triggers a cascade of events known as second messenger systems. These messengers, like runners in a relay race, carry the signal onwards, activating protein kinases. These enzymes act as switches, flipping on and off other proteins to elicit specific cellular responses.
For instance, a hormone binding to a receptor on the cell surface can initiate a signal transduction pathway that leads to increased gene expression, altering the cell’s behavior. This intricate dance of communication allows cells to adapt to their surroundings, sense changes, and maintain homeostasis.