Carbon: The Tetravalent Building Block Of Life And Organic Chemistry
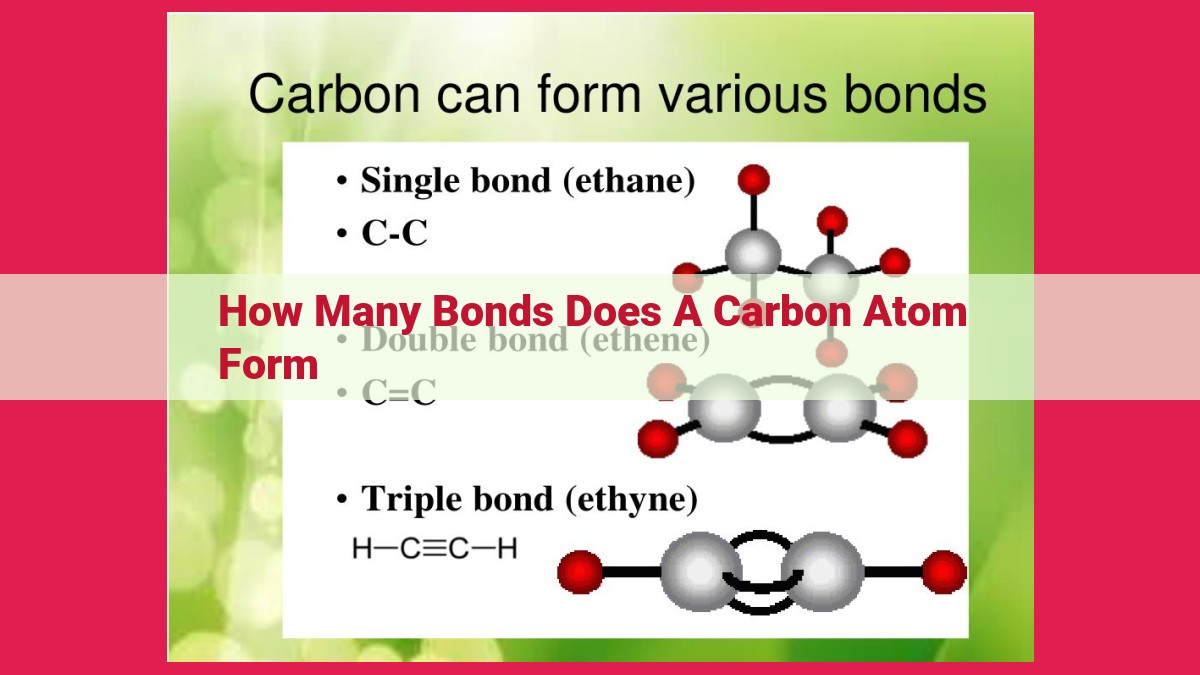
Carbon, the cornerstone of organic chemistry, exhibits remarkable bonding versatility by forming tetravalent bonds. This tetravalency stems from its four valence electrons, enabling it to form single, double, or triple bonds. These covalent bonds, formed by sharing electron pairs, determine the molecular geometry, shape, and properties of carbon compounds. The ability of carbon to form multiple bonds allows for an astonishing array of molecules, forming the basis of life and the vast diversity of organic compounds that shape our world.
Carbon’s Remarkable Bonding Capacity: The Foundation of Life
In the vast expanse of the chemical cosmos, carbon reigns supreme as the master of versatility. Its unparalleled ability to forge multiple bonds with other atoms makes it the cornerstone of life and the driving force behind the extraordinary diversity of organic molecules.
This remarkable bonding capacity stems from the very heart of carbon’s atomic structure. With four valence electrons, carbon possesses an insatiable desire to share, creating bonds that stretch beyond the confines of single connections. This remarkable trait unlocks a world of possibilities, enabling carbon to connect with itself and a multitude of other elements, forming the intricate web of molecules that define our universe.
The impact of carbon’s bonding prowess is profound. It not only shapes the structure of the molecules that make up our bodies and the world around us but also governs their properties and reactivity. From the delicate dance of DNA to the strength of steel, carbon’s versatility plays a pivotal role in shaping the very essence of life and the materials that shape our world.
Tetravalency of Carbon: The Key to Its Versatility
The remarkable versatility of carbon is largely attributed to its tetravalency, a unique property that allows it to form four covalent bonds with other atoms. This endowment plays a pivotal role in shaping the vast array of organic molecules that constitute life and nature.
The Significance of Tetravalency
Carbon’s tetravalency stems from the arrangement of its valence electrons. It possesses four valence electrons, which are the electrons in its outermost shell that determine its chemical reactivity. These valence electrons are eager to participate in covalent bonding, a process where atoms share electron pairs to attain a stable electron configuration.
Distribution of Valence Electrons
The distribution of carbon’s valence electrons is crucial for understanding its tetravalency. Carbon’s ground state electron configuration is 1s²2s²2p². The two valence electrons reside in the 2p orbitals, which are perpendicular to each other. This arrangement allows carbon to form four equivalent covalent bonds, each utilizing one of the valence electrons from the 2p orbitals.
Implications of Tetravalency
Carbon’s tetravalency has profound implications for its bonding behavior. It enables carbon to bond with a wide range of atoms, including hydrogen, oxygen, nitrogen, halogens, and even itself. This versatility allows carbon to form a staggering number of compounds with varying structures and properties. Moreover, the tetrahedral geometry of carbon-carbon bonds, resulting from sp³ hybridization, gives rise to the three-dimensional architecture of organic molecules.
In summary, the tetravalency of carbon is an indispensable characteristic that underpins its unparalleled bonding versatility and the extraordinary diversity of organic compounds that form the foundation of life and chemistry.
Beyond Single Bonds: Double and Triple Bonds – Unraveling the Wonders of Carbon’s Versatility
Carbon’s unmatched ability to form covalent bonds extends beyond its tendency to create single bonds. It can also form double and triple bonds, a testament to its remarkable versatility.
Double Bonds: A Dance of Two Shared Electron Pairs
Double bonds arise when two carbon atoms share two pairs of electrons, creating a strong and stable bond. These bonds are represented by two parallel lines in structural formulas, signifying the presence of four shared electrons. Common examples of double bonds include those found in ethene (ethylene) and butene molecules.
Triple Bonds: A Trifecta of Shared Electron Pairs
Carbon’s bonding prowess reaches its zenith with triple bonds, formed when two carbon atoms share three pairs of electrons. These extraordinarily strong bonds are indicated by three parallel lines in structural formulas. Examples of triple bonds abound in nature, such as the one found in the toxic gas acetylene.
Implications for Carbon’s Influence
The ability of carbon to form double and triple bonds has profound implications for the world we live in. These bonds provide the backbone for countless organic molecules, the building blocks of life. From the double bonds in plant chlorophyll, which facilitate photosynthesis, to the triple bonds in vitamins such as vitamin C, these bonds are essential for life’s very existence.
Unveiling the Secrets of Carbon’s Bonding Magic: The Power of Hybridization
As we delve into the fascinating world of carbon, we encounter its remarkable ability to form versatile bonds, shaping the molecular landscapes around us. At the heart of this versatility, we discover the intricate dance of atomic orbital hybridization, the key choreographer of molecular geometry.
Hybridization: A Game-Changer in Molecular Shape
Imagine the valence electrons of carbon as tiny dancers, eager to pair up and tango with other electrons. However, these dancers aren’t confined to their own space; they can fuse their energies, harmonizing into new hybrid orbitals.
The most common types of hybridization in carbon are sp3, sp2, and sp. Each hybridization type emerges from a distinctive combination of pure atomic orbitals, giving rise to specific 3D shapes.
Sp3 Hybridization: The Tetrahedral Maestro
When a carbon atom bonds with four other atoms, its sp3 orbitals come into play. These four hybrid orbitals are oriented symmetrically in a tetrahedron shape. This arrangement gives rise to molecules with bond angles of 109.5 degrees and a tetrahedral geometry, epitomized by methane (CH4).
Sp2 Hybridization: The Trigonal Planar Star
In the realm of sp2 hybridization, three hybrid orbitals form a flat, trigonal plane. The remaining unhybridized p orbital extends perpendicularly above and below the plane. This geometry manifests in molecules such as ethylene (C2H4), which exhibits bond angles of 120 degrees.
Sp Hybridization: The Linear Arrow
When a carbon atom forms a double bond, it adopts sp hybridization. In this arrangement, two hybrid orbitals align linearly, while two p orbitals remain unhybridized, perpendicular to the hybrid orbitals. This linear geometry is exemplified by molecules like acetylene (C2H2).
Molecular Geometry: Unraveling the Hidden Order in Covalent Bonding
Every molecule we encounter, from the smallest to the most complex, owes its intricate structure to the dance of electrons shared between atoms. Covalent bonding, the sharing of electron pairs, is the glue that holds molecules together. At the heart of this bonding lies carbon, an element with a remarkable ability to form multiple bonds, giving rise to a mesmerizing array of molecular geometries.
VSEPR Theory: A Blueprint for Molecular Shape
To comprehend the geometry of carbon compounds, we turn to the Valence Shell Electron Pair Repulsion (VSEPR) theory. VSEPR states that electron pairs in a molecule’s outermost shell arrange themselves in a manner that minimizes their repulsion. This arrangement dictates the molecular geometry.
Tetrahedral Geometry: A Cornerstone of Carbon Chemistry
When a carbon atom forms four single bonds, its electron pairs adopt a tetrahedral geometry. This symmetrical arrangement places the four electron pairs as far apart as possible, minimizing repulsion. Molecules with tetrahedral geometry, like methane (CH4), have four equivalent bonds pointing towards the corners of a tetrahedron.
Trigonal Planar Geometry: Flat and Symmetrical
In molecules with three bonding pairs and one lone pair (an unshared pair of electrons), the electron pairs arrange themselves in a trigonal planar geometry. The three bonding pairs form a flat equilateral triangle, while the lone pair occupies the fourth corner of the plane. An example of a molecule with trigonal planar geometry is carbon dioxide (CO2).
Linear Geometry: A Straight and Narrow Path
When a carbon atom forms two double bonds or a triple bond, its electron pairs adopt a linear geometry. This configuration minimizes repulsion by placing the electron pairs as far apart as possible along a straight line. Molecules like carbon dioxide (CO2) and acetylene (C2H2) exhibit linear geometry.
Molecular geometry plays a crucial role in understanding the properties and functions of molecules. It influences molecular polarity, reactivity, and intermolecular interactions. By unraveling the geometry of carbon compounds, we gain a deeper understanding of the vast diversity of molecules that shape our world.
Covalent Bonding: The Nature and Significance
In the realm of chemistry, carbon reigns supreme as the element of life and the foundation of countless molecules that shape our world. Its ability to form diverse bonds is the cornerstone of this versatility, enabling the creation of complex and intricate structures.
The Nature of Covalent Bonding
Covalent bonding is a type of chemical bond formed when two atoms share one or more pairs of electrons. In the case of carbon, it has four valence electrons, eagerly seeking to pair up with other atoms to form stable electron configurations.
*When carbon atoms interact, they contribute their valence electrons to form a shared pool. This electron sharing creates a covalent bond, uniting the atoms and giving rise to new molecules.
Importance in Carbon Chemistry
Covalent bonding is the dominant force that shapes the structure and properties of carbon-based compounds, known as organic molecules. These molecules are ubiquitous in living organisms, forming the building blocks of life. From proteins and DNA to carbohydrates and fats, organic molecules orchestrate the symphony of life.
Formation of a Carbon-Carbon Covalent Bond
Imagine a pair of carbon atoms, each with four valence electrons. As they approach each other, their outermost valence orbitals overlap, creating a region where their electrons can interact.
Within this overlapping region, a dance of electrons ensues. One electron from each carbon atom pairs up and spins in the same direction, forming a covalent bond. This bond becomes the foundation for the carbon-carbon backbone that characterizes organic molecules.
Comparison to Other Bonding Types
Unlike ionic bonds, where one atom donates an electron to another, *covalent bonds involve the sharing of electrons. This results in a more balanced distribution of charges and a stronger bond.
In *metallic bonds, electrons are delocalized and roam freely throughout the metal lattice, contributing to the high electrical conductivity and malleability of metals.*
The versatility of carbon’s bonding capacity has allowed life to flourish and has shaped countless aspects of our material world. This ability to form covalent bonds with other carbon atoms, as well as with hydrogen, nitrogen, and oxygen, has led to the immense diversity and complexity of organic molecules that underpin life and industry alike. Understanding the nature and significance of covalent bonding is thus essential for comprehending the fundamental principles that drive our universe.