Understanding Carbon Hybridization: Predict Hydrogen Bonding And Molecular Geometry
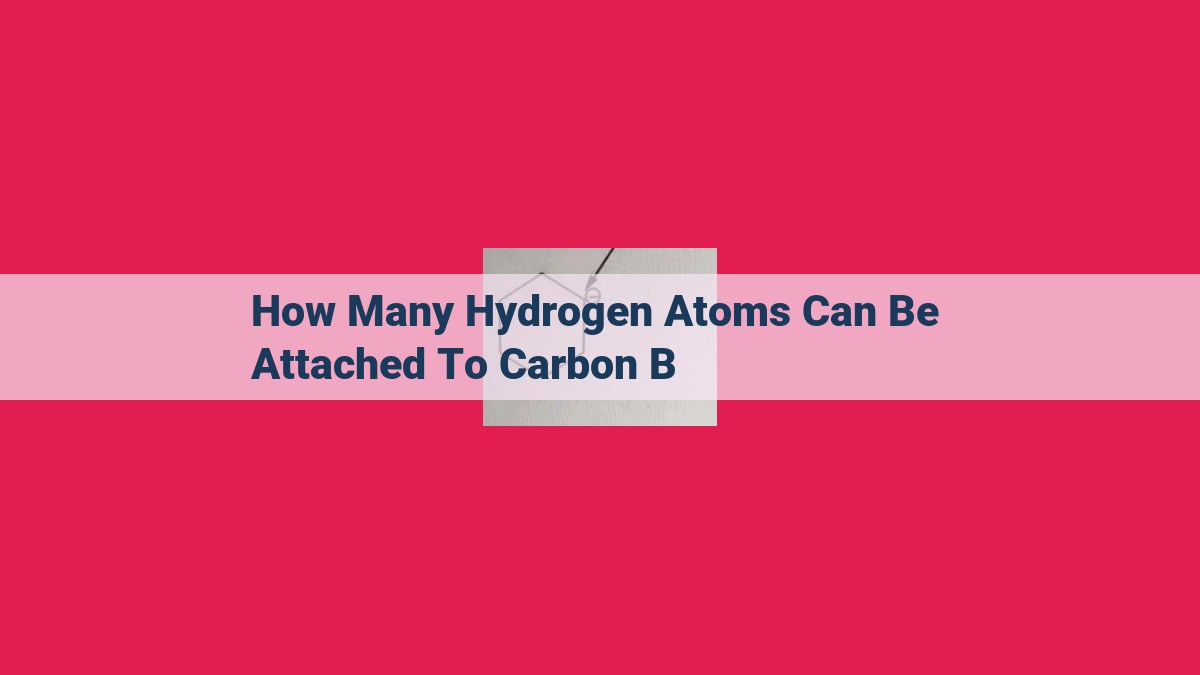
Carbon’s versatility in bonding enables it to accommodate varying numbers of hydrogen atoms, depending on its hybridization. sp3 hybridization grants carbon the ability to bond with four hydrogen atoms, forming tetrahedral molecules. sp2 hybridization allows for three hydrogen attachments, resulting in trigonal planar structures. sp hybridization limits hydrogen attachment to two, producing linear molecules. Understanding hybridization and bonding principles allows us to predict the maximum number of hydrogen atoms that can attach to carbon B, enabling accurate molecular geometry predictions.
Introduction
- Engage readers with the question of how many hydrogen atoms can attach to carbon B.
**How Many Hydrogen Atoms Can Attach to Carbon? An Atomic Adventure**
Imagine a world where atoms are like Lego blocks, each with its own unique shape and bonding capabilities. Among these atomic building blocks, carbon stands out as a true star. It’s the element that forms the backbone of life and countless other molecules. But one of its most puzzling properties is its ability to bond with various numbers of hydrogen atoms. Just how many hydrogen atoms can carbon dance with? Embark on an atomic adventure to unravel this intriguing question.
Understanding Carbon’s Chemistry
Carbon’s Valence Electrons
In the realm of chemistry, carbon stands out as a captivating element with an atomic number of 6. This means that each carbon atom harbors six valence electrons or electrons that participate in chemical bonding. These valence electrons are the key players in determining carbon’s remarkable affinity for forming bonds with other elements.
Lewis Structures
One of the most useful tools in understanding carbon’s bonding behavior is the Lewis structure. A Lewis structure depicts the arrangement of valence electrons around individual atoms, showing how they are shared to form chemical bonds. In the case of carbon, each valence electron is represented by a dot.
Hybridization
The concept of hybridization is crucial in understanding the geometry and bonding properties of carbon-containing molecules. Hybridization refers to the merging of different atomic orbitals to form hybrid orbitals that have distinct shapes and energies. These hybrid orbitals are responsible for the specific ways in which carbon atoms form bonds.
Hybridization and Bonding Capability: Unlocking Carbon’s Versatility
In the realm of chemistry, carbon stands as a true chameleon, capable of donning various “hats” known as hybridizations. This remarkable ability not only shapes its molecular structure but also dictates its bonding capacity, including the intriguing question of how many hydrogen atoms it can embrace.
sp3 Hybridization: A Tetrahedral Embrace
When carbon undergoes sp3 hybridization, it combines one s orbital and three p orbitals, resulting in four equivalent sp3 hybrid orbitals. These orbitals arrange themselves tetrahedrally, forming the foundation of countless organic molecules. Each sp3 orbital eagerly extends its “arms” to bond with four other atoms, such as four hydrogen atoms in methane (CH4).
sp2 Hybridization: Trigonal Planar Geometry
In sp2 hybridization, carbon blends one s orbital and two p orbitals, producing three sp2 hybrid orbitals. These orbitals align themselves in a trigonal planar geometry, leaving one unhybridized p orbital perpendicular to the plane. This arrangement allows carbon to form three sigma bonds (made up of sp2 orbitals) and one pi bond (formed by the unhybridized p orbital).
sp Hybridization: Linear Bonding
When carbon undergoes sp hybridization, it merges one s orbital with one p orbital, resulting in two sp hybrid orbitals oriented 180 degrees apart. These sp orbitals form linear bonds with two other atoms, such as in carbon dioxide (CO2), where carbon bonds with two oxygen atoms.
Implications for Hydrogen Attachment
The hybridization of carbon directly impacts its bonding capacity and, consequently, the number of hydrogen atoms it can attach to. In sp3 hybridization, carbon can form four sigma bonds, accommodating four hydrogen atoms as in methane. In sp2 hybridization, carbon forms three sigma bonds and one pi bond, limiting its hydrogen capacity to two, as seen in ethene (C2H4). sp hybridization restricts carbon to two sigma bonds, allowing it to bond with only one hydrogen atom, as in acetylene (C2H2).
Carbon’s ability to change its hybridization opens up a vast array of molecular possibilities. Understanding these hybridizations is crucial for deciphering the structure and properties of countless organic compounds, enabling us to harness the power of this versatile element to create new materials and advance scientific frontiers.
Sigma Bonds and Hybridization: The Key to Understanding Carbon’s Hydrogen Capacity
In the realm of chemistry, the question of how many hydrogen atoms can attach to a particular carbon atom, known as carbon B, is a captivating one. To unravel this mystery, we must delve into the fascinating world of carbon’s chemistry.
At the heart of this exploration lies the concept of hybridization. Carbon’s versatility stems from its ability to form different types of hybrid orbitals by combining its valence electrons. These orbitals govern the shape and bonding capacity of carbon, ultimately dictating how many hydrogen atoms it can embrace.
One of the most prevalent types of hybrid orbitals is the sp3 hybrid. When carbon undergoes sp3 hybridization, it merges one s orbital with three p orbitals, resulting in four equivalent sp3 hybrid orbitals. These orbitals are arranged in a tetrahedral geometry, creating an ideal platform for forming sigma bonds.
Sigma bonds are formed when the orbitals of two atoms overlap head-to-head. In the case of carbon, sp3 hybrid orbitals can engage in sigma bonding with hydrogen atoms. Each of the four sp3 orbitals has the potential to form a sigma bond, allowing carbon B to accommodate up to four hydrogen atoms.
This phenomenon is evident in compounds like methane (CH4), where carbon forms four sigma bonds with four hydrogen atoms. The symmetrical tetrahedral arrangement of the hydrogen atoms around the carbon atom reflects the sp3 hybridization and the formation of four sigma bonds.
Other types of hybridization, such as sp2 and sp, can also influence the number of hydrogen atoms that carbon B can bind. However, the relationship between hybridization and hydrogen attachment is a complex one, requiring further exploration in subsequent sections of this comprehensive guide.
**Understanding the Enigma of Pi Bonds: The Conditions for Their Formation**
Imagine two carbon atoms, each eager to share and connect. As they approach each other, they discover they possess an extra pair of electrons that crave companionship. But these electrons are not content with forming the typical head-to-head sigma bond. Instead, they soar perpendicularly, seeking a different kind of union: the alluring pi bond.
What is a Pi Bond?
Unlike sigma bonds that directly connect atoms head-on, pi bonds result from the lateral overlap of p-orbitals. These p-orbitals are dumbbell-shaped electron clouds that align sideways, creating a region of electron density above and below the atoms’ shared plane. Pi bonds are crucial in giving molecules their three-dimensional shape and stability.
Conditions for Pi Bond Formation
The formation of pi bonds is not a whimsical event; it requires specific conditions:
- ** наличието на p-orbitals:** The participating atoms must possess p-orbitals that overlap laterally to form the bond.
- Unhybridized p-orbitals: The p-orbitals must be unhybridized, meaning they have not combined with other orbitals to form hybrid orbitals.
- Parallel alignment: The p-orbitals must be aligned parallel to each other to maximize overlap.
- Adjacent atoms: The atoms must be adjacent to each other in the molecular structure, allowing their p-orbitals to effectively overlap.
Significance of Pi Bonds
Pi bonds play a critical role in the properties and behavior of molecules:
- Double bond formation: Pi bonds, in conjunction with sigma bonds, create double bonds, which are stronger and shorter than single bonds.
- Molecular geometry: Pi bonds influence the three-dimensional shape of molecules, contributing to their unique properties.
- Reactivity: Pi bonds are typically more reactive than sigma bonds due to their weaker electron density.
By understanding the conditions that enable pi bond formation, we can unlock the secrets of molecular architecture and predict the behavior of complex compounds.
Molecular Geometry and VSEPR Theory
- Introduce VSEPR theory and its use in predicting molecular geometry.
Molecular Geometry and VSEPR Theory: A Guide to Predicting Molecular Shapes
Unveiling the fascinating world of molecular geometry, we embark on a journey guided by VSEPR theory (Valence Shell Electron Pair Repulsion). This theory serves as a compass, helping us navigate the three-dimensional world of molecules by predicting their shapes.
At its core, VSEPR theory rests upon the notion that electron pairs repel each other, seeking to minimize their interactions. This repulsive force drives the electrons to arrange themselves in specific geometries that optimize their stability.
To understand molecular geometry, we must first consider the hybridization of the central atom. Hybridization involves the mixing of atomic orbitals to create new hybrid orbitals that have specific shapes and orientations. These hybrid orbitals, in turn, dictate the arrangement of electron pairs and ultimately the shape of the molecule.
For instance, in a molecule with sp³ hybridization, the four electron pairs are arranged in a tetrahedral geometry, with the central atom at the center of the tetrahedron. This tetrahedral geometry is observed in molecules such as methane (CH₄), where each carbon atom is surrounded by four hydrogen atoms.
In contrast, molecules with sp² hybridization feature a trigonal planar geometry, with the three electron pairs arranged in a flat, triangular shape. This geometry is found in molecules like ethylene (C₂H₄), where each carbon atom is bonded to two other carbon atoms and two hydrogen atoms.
Finally, molecules with sp hybridization exhibit a linear geometry, with the two electron pairs arranged in a straight line. This geometry is characteristic of molecules like carbon dioxide (CO₂), where the central carbon atom is bonded to two oxygen atoms.
By understanding the hybridization of the central atom and the repulsive forces between electron pairs, we can utilize VSEPR theory to effortlessly predict the molecular geometry of a vast array of compounds. This knowledge not only deepens our understanding of molecular structures but also serves as a foundation for exploring their chemical properties and reactivity.
Implications for Hydrogen Attachment
The number of hydrogen atoms that can attach to a carbon atom directly relates to carbon’s hybridization. Hybridization refers to the mixing of atomic orbitals to form new hybrid orbitals with different shapes and energy levels. The type of hybridization influences carbon’s bonding capacity and the geometry of the molecule it forms.
In the case of carbon, it has four valence electrons, allowing it to form a maximum of four covalent bonds. Depending on the hybridization state, carbon can have varying numbers of sigma and pi bonds, which in turn affects the number of hydrogen atoms it can attach to.
Specific Hybridizations and Hydrogen Attachment
To fully grasp the relationship between carbon’s hybridization and its ability to bind with hydrogen atoms, let’s explore specific examples of each hybridization type:
sp3 Hybridization
In sp3 hybridization, carbon’s four valence electrons hybridize to form four equivalent hybrid orbitals, each with a tetrahedral shape. This tetrahedral arrangement creates four identical sigma bonds, allowing four hydrogen atoms to attach to the carbon atom. Examples of sp3-hybridized carbon include methane (CH4), ethane (C2H6), and propane (C3H8).
sp2 Hybridization
In sp2 hybridization, three of carbon’s valence electrons hybridize to form three equivalent hybrid orbitals, each with a trigonal planar shape. The remaining unhybridized p orbital overlaps laterally with adjacent p orbitals, forming a double bond. This arrangement allows for two hydrogen atoms to attach to the carbon atom, in addition to the double bond. Examples of sp2-hybridized carbon include ethylene (C2H4), benzene (C6H6), and formaldehyde (CH2O).
sp Hybridization
In sp hybridization, two of carbon’s valence electrons hybridize to form two equivalent hybrid orbitals, each with a linear shape. The remaining two unhybridized p orbitals overlap laterally, forming a triple bond. This arrangement allows for one hydrogen atom to attach to the carbon atom, in addition to the triple bond. Examples of sp-hybridized carbon include acetylene (C2H2) and carbon monoxide (CO).