Carbon: Electron Configuration, Energy Levels, And Paramagnetism
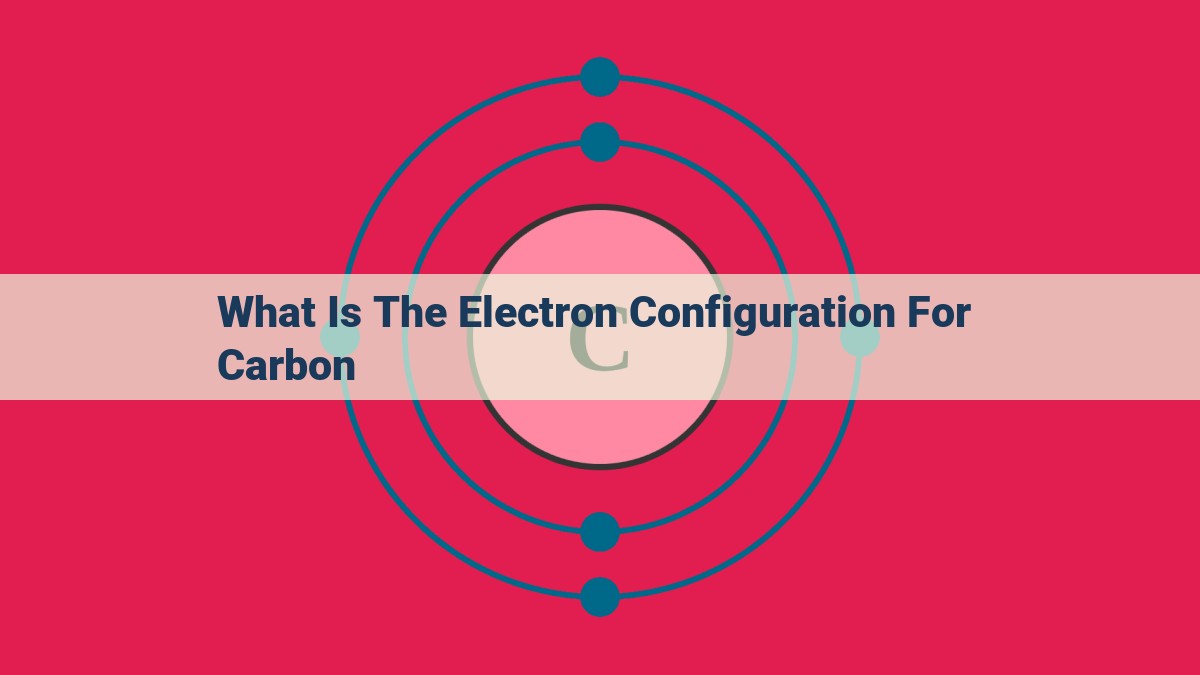
Carbon, with an atomic number of 6, possesses 6 protons in its nucleus and 6 electrons. Its three energy levels are designated as n = 1, 2, and 3. According to the Aufbau principle, electrons populate these levels in order of increasing energy. Carbon’s electron configuration is thus 1s² 2s² 2p², indicating two electrons in the 1s orbital, two in the 2s orbital, and two in the three available 2p orbitals, resulting in paramagnetism due to the parallel spins of its electrons.
Unraveling the Atomic Structure of Carbon: A Beginner’s Guide
Atomic Number and Protons: The Core of Carbon’s Identity
The atomic number, a fundamental property of every element, defines the essence of an atom. For carbon, this number is six, signifying the presence of six protons residing in its nucleus. These protons, each carrying a positive charge, form the very core of the carbon atom, establishing its unique identity.
The nucleus, the hub of the atom, also houses neutrally charged particles called neutrons, which contribute to the atom’s overall mass. Carbon’s atomic number, six, not only specifies the number of protons but also indicates the number of nucleons (the sum of protons and neutrons) in its nucleus. Understanding this fundamental aspect of carbon’s atomic structure sets the stage for exploring its intricate electronic configuration and chemical properties.
Electrons: The Building Blocks of Life
Every atom of carbon holds six electrons, tiny particles that dance around its nucleus like celestial bodies. These electrons, each carrying a negative electrical charge, are fundamental to carbon’s chemistry and life on Earth.
Electrons are not mere bystanders in the atomic realm. They play a vital role in shaping the properties of elements and determining how they interact with one another. In carbon’s case, electrons are responsible for its ability to form countless bonds, creating the vast array of organic molecules that make up living organisms.
Picture electrons as miniature planets orbiting a sun, the nucleus. They reside in specific regions of space called orbitals, each with a distinct energy level. These orbitals, named s, p, d, and f, are arranged in shells around the nucleus, with each shell corresponding to a different energy level.
Carbon’s electrons occupy the first three energy levels. Two electrons fill the innermost s orbital, another two fill the next p orbital, and the remaining two reside in the outermost p orbital. This configuration, known as electron configuration, is unique to carbon and determines its unique chemical properties.
The arrangement of electrons in orbitals is governed by a set of rules, the most important of which is the Pauli Exclusion Principle. This principle states that no two electrons can occupy the same quantum state, meaning they must differ in one or more properties, such as spin. This rule ensures that each electron has its own unique identity and prevents electrons from clumping together.
Carbon’s six electrons contribute to its ability to participate in chemical reactions. They can be transferred or shared with other atoms, forming bonds that hold molecules together. This bonding capability is the cornerstone of carbon-based life, allowing for the formation of complex and versatile structures that enable the processes of life.
Electrons are not just abstract concepts. They are the microscopic building blocks of our world, the invisible forces that shape the matter around us. By understanding the role of electrons in carbon, we gain a deeper appreciation for the intricate architecture of our universe and the fundamental nature of life itself.
Energy Levels and Quantum States: Delving into Carbon’s Atomic Structure
In the realm of chemistry, understanding the atomic structure of elements is paramount. Carbon, an essential building block of life, exhibits a unique atomic structure that dictates its remarkable properties. One crucial aspect of carbon’s atomic structure is its energy levels and quantum states.
Energy Levels: The Orbital Highway
Imagine carbon’s nucleus as a bustling metropolis, surrounded by a series of concentric rings known as energy levels. These energy levels are designated by the principal quantum number (n). Carbon possesses three distinct energy levels, each representing a different energy range. The first energy level (n = 1) is closest to the nucleus, followed by the second (n = 2) and third (n = 3).
Quantum States: The Electron’s Dwelling
Within each energy level reside compartments called orbitals, which act as the electron’s abode. Orbitals are characterized by the azimuthal quantum number (l), which denotes their shape and orientation. Carbon’s first energy level contains a single 1s orbital, shaped like a sphere. The second energy level comprises two orbitals: a 2s orbital resembling a larger sphere and three 2p orbitals, which take on dumbbell-shaped geometries.
The Quantum Maze: Navigating Energy Levels
Electrons, the negatively charged particles that orbit the nucleus, occupy these orbitals in accordance with specific rules. The Aufbau principle dictates that electrons fill orbitals in order of increasing energy, starting with the lowest energy level. Consequently, carbon’s six electrons distribute as follows: two in the 1s orbital, two in the 2s orbital, and two in the 2p orbitals.
Hund’s Rule: The Electron’s Dance
The Hund’s rule governs the spin of electrons within orbitals. It states that electrons prefer to occupy separate orbitals with parallel spins. This phenomenon explains carbon’s paramagnetic properties, as its two 2p electrons possess unpaired spins, making it susceptible to the influence of magnetic fields.
Pauli’s Principle: The Electron’s Identity
The Pauli exclusion principle ensures that no two electrons can occupy the same quantum state. This principle safeguards the distinct identity of each electron, preventing them from merging into an indistinguishable mass.
The Significance of Energy Levels and Quantum States
Understanding the energy levels and quantum states of carbon provides a solid foundation for comprehending its chemical behavior. The arrangement and properties of these orbitals govern carbon’s ability to form bonds with other atoms, giving rise to the vast array of molecules that form the backbone of our natural world.
Orbitals and Angular Momentum: Delving into the Quantum Realm of Carbon
In the fascinating realm of quantum mechanics, electrons don’t merely dance around the nucleus in circular paths. Instead, they occupy specific regions called orbitals, each characterized by its unique shape and energy level. These orbitals are like tiny energy pockets that electrons can inhabit, much like rooms in a house.
Carbon, with its six electrons, has a total of three energy levels (n=1, 2, and 3). Each energy level can accommodate a specific number of orbitals, which are further classified into sublevels based on their angular momentum. Angular momentum refers to the amount of spinning and swirling that electrons do within an orbital.
The sublevels are labeled as s, p, d, and f. Each energy level has a different set of sublevels, and each sublevel can hold a specific number of electrons.
First Energy Level (n=1)
The first energy level, with its sphere-like s orbital, can hold a maximum of two electrons. In carbon, this orbital is filled with two electrons, represented as 1s².
Second Energy Level (n=2)
The second energy level has a more complex structure. It consists of one s orbital and three p orbitals. The s orbital can hold two electrons, while each p orbital can accommodate two electrons with opposite spins. This level is also fully occupied in carbon, with its configuration as 2s² 2p².
The three p orbitals are oriented along the three axes (x, y, and z) in space, giving them directional properties. They are often visualized as dumbbells or teardrop shapes.
Electrons as Miniature Spinning Magnets
Electrons are more than just negatively charged particles. They also possess an intrinsic property known as spin. Imagine electrons as miniature spinning magnets, with their north and south poles. Hund’s rule, a fundamental principle in quantum mechanics, dictates that electrons in the same orbital must have parallel spins. This means that the tiny magnets align their north and south poles in the same direction.
Pauli Exclusion Principle: No Two Electrons Alike
The Pauli exclusion principle is the ultimate guarantor of uniqueness in the quantum world. It states that no two electrons can occupy the same quantum state. A quantum state is a combination of energy level, sublevel, and spin. Thus, in the world of electrons, every quantum state is like a unique fingerprint, preventing any two electrons from being identical.
This intricate dance of electrons within orbitals, governed by the principles of angular momentum, spin, and the Pauli exclusion principle, is what ultimately defines the electronic structure of carbon and its unique chemical properties.
The Aufbau Principle: Understanding Carbon’s Electron Configuration
In the vibrant tapestry of the atomic world, carbon stands out as a versatile element, its unique properties shaping the very fabric of life. To unravel the secrets of carbon’s extraordinary nature, we delve into its innermost sanctum: the electron cloud that orbits its nucleus.
The Aufbau principle, a guiding light in the realm of quantum mechanics, dictates the orderly filling of these electron orbitals. Each electron seeks its most comfortable abode, aligning itself in a specific energy level and orbital. The energy levels are numbered (n) in ascending order from the nucleus, and each level can accommodate a finite number of electrons.
For carbon, with its atomic number of 6, the Aufbau principle guides electrons to occupy the lowest energy levels first. The innermost 1s orbital welcomes two electrons, forming a stable foundation for the electron cloud. The next two electrons find their niche in the 2s orbital, aligning themselves with the first two.
The remaining two electrons embark on a slightly more energetic adventure, settling into the 2p orbitals. Unlike the s orbitals which are spherical in shape, p orbitals are elongated and oriented along the x, y, and z axes. Each of these three p orbitals accommodates a single electron, giving carbon a total of six electrons in its outermost energy level.
The electron configuration of carbon can thus be summarized as 1s² 2s² 2p². This notation signifies the two electrons in the 1s orbital, two electrons in the 2s orbital, and two electrons distributed among the three 2p orbitals.
Hund’s Rule and Electron Spin: Unraveling Carbon’s Magnetic Personality
In the realm of atomic structure, the behavior of electrons plays a crucial role in defining an element’s unique characteristics. For carbon, the sixth element in the periodic table, its magnetic properties are shaped by the enigmatic interplay of its electron spins, a concept governed by Hund’s rule.
Hund’s rule states that, when filling orbitals of the same energy level, electrons prefer to have parallel spins. This means that rather than pairing up and occupying the same orbital, they align their spins in the same direction. This phenomenon is driven by a subtle energy preference that results from the repulsive forces between electrons with the same spin.
In the case of carbon, its 2p² configuration involves two electrons in the 2p sublevel. According to Hund’s rule, these electrons will initially occupy separate orbitals with parallel spins. This results in a net magnetic moment for the carbon atom due to the unpaired spins. The presence of unpaired electrons makes carbon paramagnetic, meaning it is attracted to magnetic fields.
The significance of Hund’s rule extends beyond its impact on carbon’s magnetic behavior. This principle is essential in understanding the electronic configurations of all elements, as it governs the filling of orbitals and determines the overall energy state of the atom. By unraveling the mysteries of electron spin, Hund’s rule provides a deeper understanding of the fundamental building blocks of matter.
The Pauli Exclusion Principle and Electron Uniqueness
In the realm of quantum mechanics, the Pauli exclusion principle reigns supreme, ensuring the distinct identity of every electron. This fundamental principle states that no two electrons in an atom can have the same set of quantum numbers, which include energy level, sublevel, and spin.
Imagine electrons as energetic dancers, each with a unique dance style. The Pauli exclusion principle dictates that these dancers cannot perform the same dance steps at the same time. Each electron must have its own exclusive stage, its own energy level, and its own sublevel.
This principle plays a crucial role in shaping the structure of atoms. It determines the number of electrons that can occupy each energy level and sublevel. For instance, the first energy level (n=1) can hold a maximum of two electrons in the 1s sublevel. The second energy level (n=2) can accommodate eight electrons, with two in the 2s sublevel and six in the 2p sublevel.
Moreover, the Pauli exclusion principle governs the phenomenon of electron spin. Electrons are like tiny magnets, and their spin can be either “up” or “down.” The principle dictates that each orbital can hold a maximum of two electrons, with opposite spins. This spin opposition ensures that electrons minimize their repulsion and maintain a stable atomic structure.
The Pauli exclusion principle is not merely a theoretical concept; it has profound implications in our everyday lives. It underlies the behavior of electrons in semiconductors, the basis of modern electronics. It also explains the periodic table of elements, as the number of electrons in each element determines its chemical properties.
In summary, the Pauli exclusion principle is the cornerstone of electron behavior. It ensures that electrons maintain their individuality, orchestrate the formation of atoms, and underpin the technological marvels of the 21st century.