Understanding Carbon’s Bonding Properties For Stable Molecular Formations
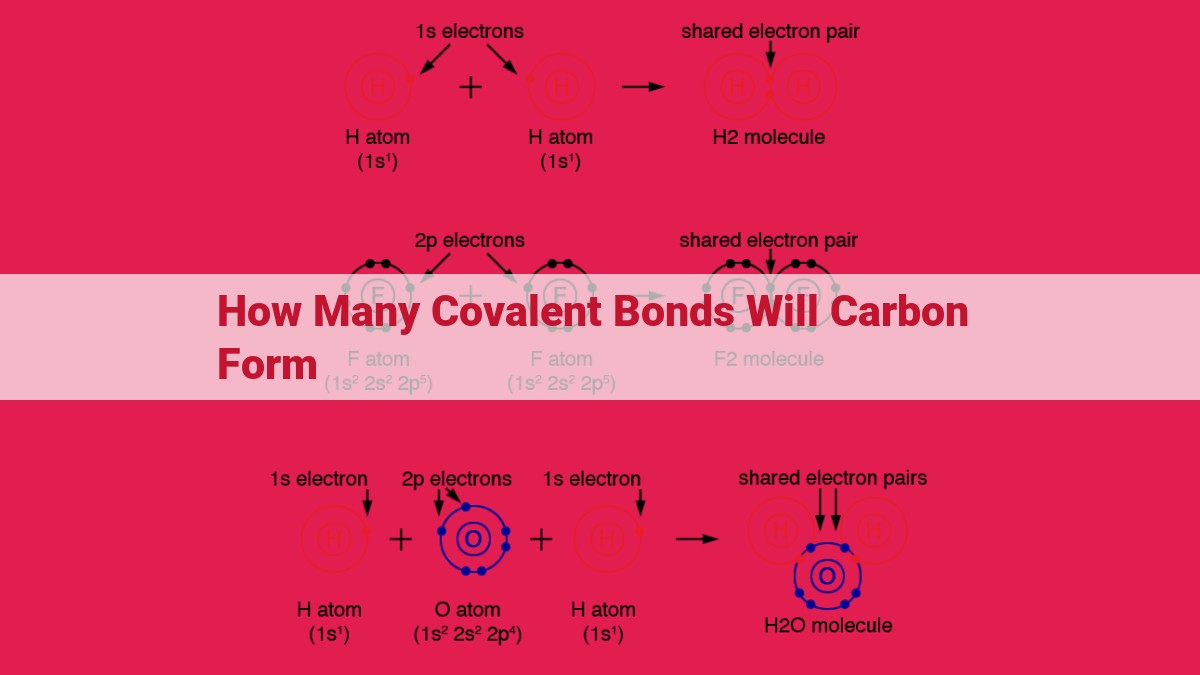
Carbon forms covalent bonds to attain a stable octet of valence electrons. Through hybridization, carbon combines its atomic orbitals to form hybrid orbitals, which determine the molecular shape. With four valence electrons, carbon can form four covalent bonds, allowing it to share electrons with other atoms to achieve the desired electron configuration and form stable compounds.
Hybridization: Unveiling the Dance of Electron Pairs
In the atomic realm, electrons don’t simply float around the nucleus like celestial bodies. Instead, they dance within designated areas called orbitals. But sometimes, these orbitals aren’t content with their solitary existence. They merge, intertwining like graceful partners, to form something new: hybrid orbitals.
This hybridization is not a mere coincidence. It’s a delicate balancing act, where atomic orbitals sacrifice their individual identities to create a new set of hybrid orbitals with tailored characteristics. Each hybrid orbital is a unique blend of the parent orbitals, inheriting their energy and spatial orientation.
The significance of hybridization extends beyond its theoretical implications. It holds the key to understanding the molecular shape, the three-dimensional arrangement of atoms within a molecule. Hybrid orbitals dictate the angles between atoms, giving molecules their distinctive shapes. For example, methane (CHâ‚„) has a tetrahedral shape because the carbon atom’s four valence electrons occupy four equivalent hybrid orbitals, forming bonds with hydrogen atoms at the vertices of a tetrahedron.
Comprehending hybridization is essential for unlocking the secrets of molecular structure and understanding the countless chemical interactions that shape our world. It’s a testament to the intricate dance of electrons, where seemingly abstract concepts translate into tangible, observable properties that govern the behavior of matter.
Valence Electrons: The Gateway to Chemical Bonding
In the fascinating world of chemistry, the dance of electrons defines how atoms interact and form the building blocks of our existence. Among these electrons, a special group known as valence electrons holds the key to understanding the captivating process of chemical bonding.
Valence electrons are those that reside in the outermost energy level of an atom. They are the free spirits of the atomic world, eager to explore and bond with their neighbors. The number of valence electrons an atom possesses significantly influences its chemical properties and reactivity.
Let’s turn our attention to the element carbon, the versatile foundation of life on Earth. Carbon boasts four valence electrons, eager to participate in the dance of chemical bonding. This quartet of electrons empowers carbon to form a multitude of compounds, giving rise to the dazzling diversity of organic molecules that grace our world.
Valence electrons are the driving force behind chemical bonding, the process that holds atoms together and creates the countless compounds we encounter in our daily lives. As atoms seek stability, they strive to acquire a complete set of valence electrons, either by gaining or losing electrons. This quest for a stable electron configuration is the essence of chemical bonding.
So, there you have it, a glimpse into the world of valence electrons, the maestros of chemical bonding. Their dance orchestrates the formation of molecules, paving the way for the intricate tapestry of life and chemistry.
The Octet Rule: A Blueprint for Stability
In the realm of chemistry, electrons play a pivotal role in determining the behavior and properties of atoms and molecules. Among these, valence electrons stand out as the key players in chemical bonding. They are the outermost electrons in an atom, eager to participate in the intricate dance of bonding.
The octet rule serves as a guiding principle in chemistry, dictating that atoms strive to achieve a stable configuration of eight valence electrons in their outermost shell. This rule holds immense relevance to carbon, the foundation of all organic molecules. Carbon, with its six valence electrons, falls just two shy of the coveted octet.
To attain stability, carbon embarks on a journey of gaining or losing electrons. It has two main options:
-
Gaining two electrons: This transforms carbon into a carbide ion (C-), which now boasts a stable octet.
-
Losing four electrons: By doing so, carbon becomes a carbonium ion (C+), also achieving an octet configuration.
The choice between gaining or losing electrons depends on the surrounding chemical environment. In the presence of more electronegative elements, such as oxygen, carbon tends to lose electrons to form covalent bonds, sharing electrons with other atoms to reach a stable octet.
Covalent Bonds: The Shared Electron Economy
- Explain the formation of covalent bonds by sharing valence electrons.
- Determine the number of covalent bonds carbon can form.
Covalent Bonds: **The Shared Electron Economy
In the realm of chemistry, certain atoms have a captivating ability to form partnerships, not unlike the enduring bonds of friendship. These partnerships, known as covalent bonds, are forged through the sharing of valence electrons. Valence electrons are the outermost electrons in an atom’s orbit, and they play a crucial role in determining the atom’s chemical properties.
Carbon, the versatile building block of life, stands out with its four valence electrons. This sets the stage for carbon to form an impressive number of covalent bonds. It can share its valence electrons with other atoms, creating strong and stable compounds.
The process of forming a covalent bond is akin to a dance between atoms. Each atom contributes one or more valence electrons, which then join forces to form a shared pair of electrons. This shared pair creates a molecular orbital, which is a region of space where the electrons are most likely to be found. The covalent bond, like a sturdy bridge, holds the atoms together by the mutual attraction between their positively charged nuclei and the negatively charged shared electrons.
The number of covalent bonds that carbon can form is directly related to the number of valence electrons it possesses. With four valence electrons, carbon can form four covalent bonds. This versatility allows carbon to connect with a wide range of other atoms, giving rise to the countless organic molecules that make up the world around us.
Lewis Symbols: Deciphering Valence Electrons Visually
In the captivating world of chemistry, Lewis symbols emerge as a powerful tool to unravel the secrets of valence electrons, those crucial players in the realm of bonding. These symbols, named after the renowned chemist G.N. Lewis, provide a visual representation of the number and arrangement of valence electrons surrounding an atom.
Each element has a unique Lewis symbol, which resembles a dot diagram. The dots represent valence electrons, the electrons that determine an atom’s chemical reactivity. For instance, the Lewis symbol of carbon is:.
:C:
This symbol indicates that carbon has four valence electrons. These electrons are arranged as two pairs, represented by the two dots on each side of the carbon atom.
Lewis symbols not only reveal the number of valence electrons but also predict the types of bonds an atom can form. Atoms strive to achieve a stable octet of valence electrons, which corresponds to the electron configuration of the nearest noble gas. Carbon has four valence electrons, so it aims to gain or lose four electrons to attain this stable configuration.
By studying Lewis symbols, we can gain insights into the electronic structure of atoms and predict their chemical behavior. These symbols serve as a visual roadmap, guiding our understanding of the intricate world of chemical interactions.
Structural Formulas: Unveiling the Molecular Architecture
In the realm of chemistry, structural formulas play a pivotal role in understanding the intricate connectivity of atoms within molecules. These formulas serve as a visual representation, providing a window into the molecular architecture that governs the behavior and properties of substances.
Imagine a molecule as a miniature construction set, with atoms serving as the building blocks and bonds acting as the connectors. Structural formulas capture this arrangement by employing lines to represent the bonds and symbols to denote the atoms. By deciphering these formulas, we gain insights into the shape, bonding patterns, and reactivities of molecules.
In the case of carbon chains, a series of carbon atoms linked together, structural formulas reveal the sequence and connectivity of these atoms. For example, the structural formula of ethane, a two-carbon molecule, is CH3-CH3. This formula tells us that each carbon atom is bonded to three hydrogen atoms, and the two carbon atoms are connected by a single bond.
By interpreting structural formulas, we not only comprehend the connectivity of atoms but also lay the foundation for understanding the physical and chemical properties of molecules. They empower chemists to predict the reactivity of a substance, its melting and boiling points, and even its interactions with other molecules.
Unveiling the Influence of Hybridization on Molecular Shape
In the realm of chemistry, understanding the intricacies of electron behavior is crucial to unraveling the secrets of molecular architecture. Hybridization, a fundamental concept, holds the key to deciphering the captivating dance of electrons and their profound impact on the shape of molecules.
Imagine the electron cloud surrounding an atom as a collection of distinct orbitals, each shaped like dumbbells or spheres. When these orbitals undergo hybridization, they magically merge their identities, forming new hybrid orbitals. These hybrid orbitals, adorned with unique shapes and orientations, dictate the spatial arrangement of atoms within a molecule, ultimately determining its overall shape.
Consider the ubiquitous element carbon, the building block of life. Its quest for stability drives it to adopt a tetrahedral-shaped electron cloud, courtesy of its sp3 hybridization. This hybridization bestows upon carbon the ability to form four covalent bonds, each directed towards the vertices of a tetrahedron.
Sp3 hybridization, a symphony of merging orbitals, gives rise to four equivalent hybrid orbitals, each containing a single unpaired electron. These hybrid orbitals then engage in a grand waltz, sharing their electrons with neighboring atoms to form covalent bonds. The resulting molecule assumes a tetrahedral geometry, with the four bonded atoms occupying the four corners of the tetrahedron.
Thus, the hybridization of atomic orbitals serves as the maestro, orchestrating the molecular dance. It governs the shape and bonding behavior of molecules, dictating their properties and paving the way for the diverse tapestry of chemical compounds that shape our world.