Cam Photosynthesis: An Adaptation To Minimize Photorespiration In Arid Environments
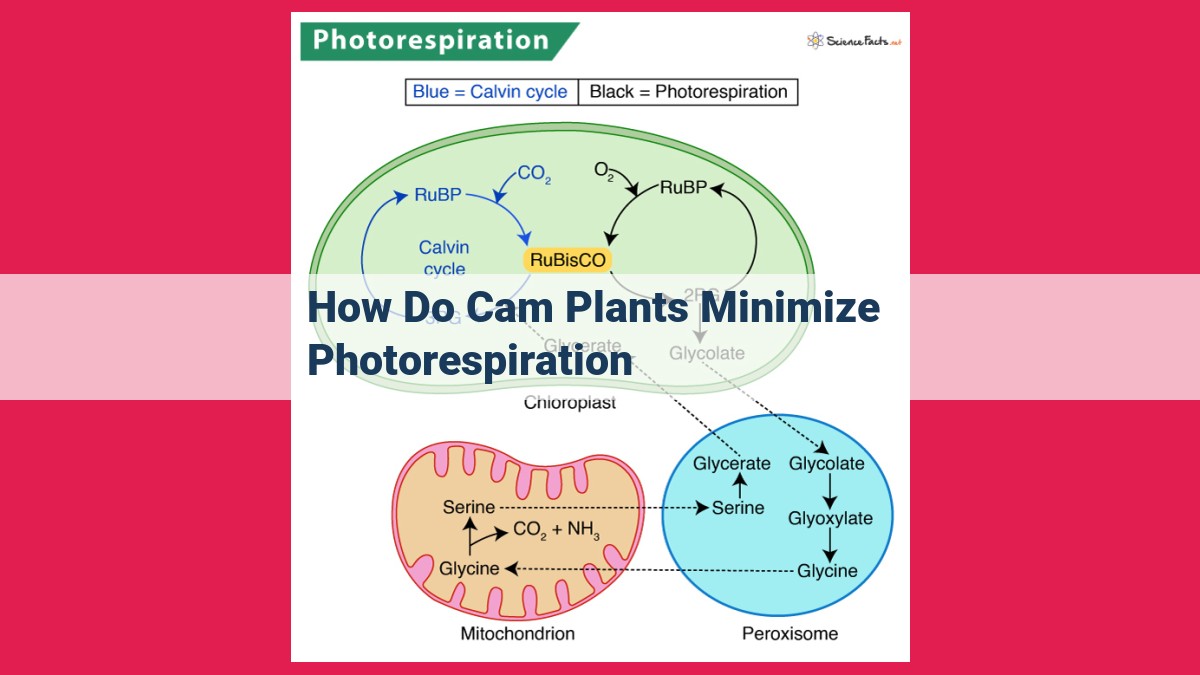
CAM plants employ a unique photosynthetic adaptation to minimize photorespiration. They separate CO2 fixation and release temporally: at night, they open their stomata to absorb CO2 and convert it into organic acids via PEP carboxylase. During the day, they close their stomata to conserve water and release CO2 from the organic acids through NADP-malic enzyme. This separation prevents the interaction of Rubisco with oxygen, reducing photorespiration and maximizing carbon assimilation efficiency in arid environments.
How CAM Plants Minimize Photorespiration
In the world of plants, there exists a fascinating adaptation known as Crassulacean Acid Metabolism (CAM). CAM plants possess a unique photosynthetic strategy that allows them to thrive in arid and water-scarce environments where other plants would struggle to survive. One of the key benefits of CAM is its ability to minimize photorespiration, a process that can significantly reduce plant growth and productivity.
Photorespiration is a wasteful metabolic pathway that occurs in plants when they are exposed to high levels of oxygen and low levels of carbon dioxide. During photorespiration, plants consume valuable energy and release carbon dioxide into the atmosphere, leading to a decrease in overall carbon fixation and growth. CAM plants have evolved to overcome this challenge by separating the processes of carbon dioxide fixation and release into two distinct phases – nighttime and daytime.
At night, when temperatures are cooler and water loss is minimized, CAM plants open their stomata (pores on their leaves) to absorb carbon dioxide from the air. This carbon dioxide is then converted into organic acids by a key enzyme called PEP carboxylase. These organic acids are stored in the plant’s vacuoles until the following day.
During the day, when temperatures rise and water loss becomes a concern, CAM plants close their stomata to conserve water. Inside the plant, the organic acids stored from the previous night are broken down by an enzyme called NADP-malic enzyme, releasing carbon dioxide within the leaf. This carbon dioxide is then used in photosynthesis to produce sugars, the energy currency of plants.
By separating carbon dioxide fixation and release into distinct phases, CAM plants are able to minimize photorespiration and maximize carbon fixation efficiency. This adaptation allows them to thrive in environments where water availability is limited and carbon dioxide levels are low, making them important players in the ecology of arid regions.
Crassulacean Acid Metabolism (CAM): A Plant’s Secret Weapon Against Photorespiration
Crassulacean Acid Metabolism (CAM) is an extraordinary adaptation that allows certain plants to thrive in harsh, arid environments. Unlike most plants, CAM plants have evolved a unique two-step photosynthetic process to minimize the energy-wasting process known as photorespiration.
During the night, CAM plants open their stomata, tiny pores on their leaves, to absorb carbon dioxide (CO2). This CO2 is then fixed into organic acids, primarily malic acid, by an enzyme called PEP carboxylase. These organic acids are stored in the plant’s vacuoles until the following day.
At dawn, CAM plants close their stomata to conserve water. They then break down the stored organic acids in their chloroplasts, releasing CO2 into the surrounding atmosphere. This CO2 is then used for photosynthesis, the process by which plants convert light energy into sugars.
The Two-Step CAM Process
The CAM process is divided into two distinct phases:
Nighttime Phase
- Stomata open to absorb CO2.
- PEP carboxylase enzyme fixes CO2 into organic acids (malic acid).
Daytime Phase
- Stomata close to conserve water.
- NADP-malic enzyme enzyme breaks down organic acids, releasing CO2.
- Released CO2 is used for photosynthesis.
CAM vs. C3 and C4 Photosynthesis
Unlike C3 and C4 plants, which perform CO2 fixation and release simultaneously, CAM plants temporally separate these processes. This separation allows CAM plants to minimize photorespiration, a process that releases CO2 and depletes plant energy.
Ecological Significance of CAM
CAM is particularly advantageous in arid environments where water is scarce. By closing their stomata during the day, CAM plants minimize water loss through transpiration. This adaptation allows them to survive and thrive in harsh conditions where other plants cannot.
Nighttime CO2 Fixation in CAM Plants
Crassulacean acid metabolism (CAM) is a unique photosynthetic adaptation that allows certain plants to survive in arid environments where water is scarce. One of the key strategies employed by CAM plants to minimize water loss is the temporal separation of CO2 fixation and release.
Stomata Open for CO2 Absorption
Unlike other plants, CAM plants open their stomata at night instead of during the day. This nighttime opening allows them to absorb CO2 from the atmosphere while minimizing water loss through transpiration. The stomata remain closed during the day to reduce water evaporation.
The Role of PEP Carboxylase
Once CO2 enters the plant, it is converted into organic acids through a process called PEP carboxylation. This reaction is catalyzed by an enzyme known as PEP carboxylase. The organic acids produced, such as malate, are stored in the plant’s vacuoles until they are needed.
**Daytime CO2 Release: CAM’s Water-Saving Strategy**
As the sun rises, CAM plants undergo a remarkable physiological transformation. Their stomata, tiny pores on their leaves that allow gas exchange, remain tightly closed during the daylight hours. This adaptation significantly reduces water loss through transpiration, helping the plant conserve precious moisture in arid environments.
Despite the closure of their stomata, CAM plants continue to photosynthesize. The key to this efficiency lies in NADP-malic enzyme, an essential enzyme responsible for breaking down the organic acids produced during the previous night’s CO2 fixation.
During the day, NADP-malic enzyme breaks down these organic acids, releasing CO2 and simultaneously generating NADPH and ATP, two energy-rich molecules. The released CO2 is then used for photosynthesis, providing the necessary carbon source for the plant’s growth and development.
This ingenious process allows CAM plants to bypass the wasteful photorespiration cycle, which consumes energy and carbon without providing a net benefit to the plant. By temporally separating CO2 fixation and release, CAM plants effectively minimize photorespiration and maximize their photosynthetic efficiency, enabling them to thrive in dry and harsh environments where water conservation is paramount.
Enzymes Involved in CAM
The intricate machinery of CAM photosynthesis relies on specific enzymes to execute its unique two-step process. Let’s delve into the critical roles played by two key enzymes: PEP carboxylase and NADP-malic enzyme.
PEP Carboxylase: The Nighttime CO2 Fixer
As night falls, CAM plants unfurl their stomata, inviting CO2 to enter. PEP carboxylase, an enzyme residing in the cytosol, is the master of capturing this precious gas. It catalyzes the conversion of phosphoenolpyruvate (PEP) into oxaloacetate, trapping CO2 into organic acids. These organic acids, such as malate and aspartate, serve as temporary reservoirs of fixed carbon.
NADP-Malic Enzyme: The Daytime CO2 Liberator
With the dawn of a new day, CAM plants seal their stomata, conserving water while their stomata remain closed. NADP-malic enzyme, an enzyme located in the chloroplasts, takes center stage. It orchestrates the breakdown of the organic acids accumulated during the night, releasing CO2 and regenerating PEP. This released CO2 is then utilized by RuBisCO, the enzyme responsible for carbon fixation in photosynthesis.
The Interplay of Enzymes in CAM
The synchronized action of PEP carboxylase and NADP-malic enzyme is central to CAM’s success. PEP carboxylase captures CO2 at night, protecting it from photorespiration. NADP-malic enzyme releases CO2 during the day, allowing it to be recaptured by RuBisCO. This temporal separation of CO2 fixation and release ensures that photorespiration, which wastes precious carbon and energy, is minimized.
CAM vs. C3 and C4 Photosynthesis: A Tale of Carbon Fixation and Release
In the realm of plant physiology, carbon fixation is a crucial process that allows plants to harness the sun’s energy and convert it into food. Among the various photosynthetic pathways, CAM (Crassulacean Acid Metabolism) stands out as a unique adaptation that enables certain plants to thrive in arid environments by minimizing photorespiration.
Distinct Carbon Fixation Pathways
In contrast to C3 and C4 plants, CAM plants employ a two-step process to fix carbon dioxide. During the night, when temperatures are cooler and water vapor loss is reduced, CAM plants open their stomata to absorb CO2. This CO2 is then converted into organic acids by PEP carboxylase, an enzyme that plays a vital role in carbon fixation.
During the daytime, CAM plants close their stomata to conserve water. Simultaneously, an enzyme known as NADP-malic enzyme breaks down the organic acids produced during the night, releasing CO2. This released CO2 is then used in a second round of carbon fixation, reducing the risk of photorespiration.
Temporal Separation: A Key to Minimizing Photorespiration
Photorespiration is a wasteful process that consumes energy and reduces plant productivity. However, CAM plants have evolved a clever strategy to minimize photorespiration. By temporally separating carbon fixation and release, CAM plants ensure that the CO2 released during the day is immediately utilized for photosynthesis, preventing its loss through photorespiration.
Ecological Significance of CAM
The ability to minimize photorespiration provides CAM plants with a significant advantage in arid environments. By conserving water and reducing energy waste, CAM plants can thrive where other plants struggle to survive. This adaptation has allowed CAM plants to colonize diverse habitats, including deserts, savannas, and grasslands.
CAM photosynthesis is a fascinating adaptation that allows certain plants to flourish in harsh environments by minimizing photorespiration. Through a unique two-step process and temporal separation of carbon fixation and release, CAM plants have evolved a remarkable strategy that optimizes their photosynthetic efficiency and enhances their survival in water-limited conditions.