Calculating Electrochemical Cell Potential: A Step-By-Step Guide To Determine E° Cell
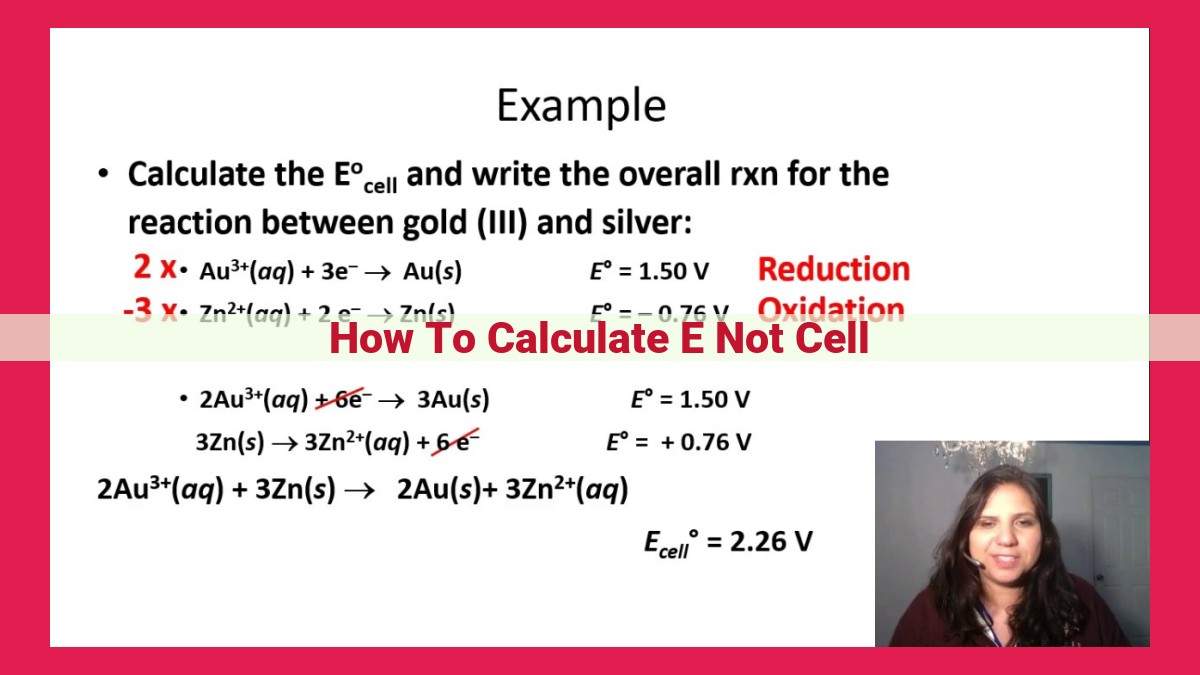
To calculate E° cell, the electromotive force of an electrochemical cell, use the standard reduction potentials from a table. Subtract the standard reduction potential of the anode (oxidation half-cell) from the standard reduction potential of the cathode (reduction half-cell). This difference represents the overall driving force of the reaction and gives you the E° cell.
Understanding Electromotive Force (EMF): The Key to Unlocking Electrochemical Reactions
Embark on a Scientific Journey
Prepare yourself for an electrifying adventure as we delve into the captivating world of electrochemistry. Today’s voyage will focus on a crucial concept: electromotive force (EMF) – the driving force behind electrochemical cells.
EMF, measured in volts, is the electrical potential difference between two electrodes in an electrochemical cell. It plays a pivotal role in determining the spontaneity and efficiency of electrochemical reactions.
Electrochemical Cells: A Tale of Two Halves
Electrochemical cells, like tiny powerhouses, consist of two half-cells. Each half-cell houses an electrode submerged in an electrolyte solution. These half-cells, when connected by a metal wire and a salt bridge, create a pathway for electrons to flow. One half-cell undergoes oxidation (loss of electrons), while the other undergoes reduction (gain of electrons).
Standard Reduction Potentials: A Glimpse into the Redox World
To quantify the tendency of half-cells to undergo reduction, scientists introduced the concept of standard reduction potential. This value, measured in volts, indicates the potential difference between a half-cell and a standard hydrogen electrode (SHE). A positive standard reduction potential signifies a higher tendency for reduction, while a negative value indicates a preference for oxidation.
The Nernst Equation: Unveiling the Effects of Concentration
The Nernst equation, a powerful tool in electrochemistry, allows us to calculate the reduction potential of a half-cell under non-standard conditions, where concentrations may vary. By considering the impact of concentration on electrical potential, we gain a deeper understanding of electrochemical systems.
Standard Cell Potential: The Heartbeat of Electrochemical Reactions
Standard cell potential, the algebraic sum of the standard reduction potentials of the two half-cells, is a fundamental property of electrochemical cells. It determines the overall spontaneity and maximum electrical work that can be extracted from the reaction. A positive standard cell potential indicates a spontaneous reaction, while a negative value suggests the need for an external energy input.
Voltaic and Electrolytic Cells: The Two Faces of Electrochemistry
Electrochemical cells come in two main flavors: voltaic and electrolytic. Voltaic cells, like tiny batteries, harness spontaneous reactions to generate electricity. Electrolytic cells, on the other hand, require an external electrical input to drive non-spontaneous reactions, such as the electrolysis of water.
Electromotive force (EMF) lies at the heart of electrochemistry, enabling us to predict and control electrochemical reactions. The Nernst equation and standard reduction potential tables empower scientists and engineers to design efficient electrochemical systems for a myriad of applications, from energy storage to industrial processes.
Introduce the equation for calculating E° cell.
Unveiling the Secrets of Electrochemical Cells: A Guide to Calculating E° Cell
Imagine yourself as an intrepid explorer embarking on a journey into the realm of electrochemistry. Your mission? To conquer the enigmatic concept of electromotive force (EMF) and master the skill of calculating E° cell, the key to understanding the behavior of electrochemical cells.
In this electrifying adventure, we’ll delve into the fascinating world of half-cells, the building blocks of electrochemical cells. We’ll encounter the standard reduction potential and the standard hydrogen electrode (SHE), two crucial reference points in electrochemistry.
Along the way, we’ll uncover the secrets of the Nernst equation, a powerful tool that allows us to calculate the reduction potential of half-cells under various conditions. This equation is like a magic wand, transforming non-standard situations into understandable realms.
Now, let’s confront the heart of our quest: the standard cell potential (E° cell). This enigmatic value holds the key to understanding the spontaneous nature of electrochemical reactions and predicting the direction of electron flow. We’ll explore the equation that connects E° cell to standard reduction potentials and witness the transformative power of these tables.
Finally, we’ll venture into the thrilling worlds of voltaic and electrolytic cells, two sides of the electrochemical coin. We’ll unravel the mysteries of spontaneous and non-spontaneous reactions, and discover the crucial role of EMF in voltaic cells and applied potential in electrolytic cells.
Armed with these newfound powers, you’ll emerge as a seasoned electrochemist, ready to conquer any electrochemical challenge that comes your way. So, let’s embark on this electrifying journey and unravel the secrets of E° cell together!
Calculating E° Cell: A Step-by-Step Guide
In the realm of electrochemistry, understanding how to calculate the electromotive force (EMF) of a cell is crucial for deciphering electrochemical reactions. EMF, measured in volts, quantifies the electrical potential difference between two electrodes in an electrochemical cell. It serves as a driving force, dictating the direction and spontaneity of the chemical reactions taking place within the cell.
Half-Cells: The Building Blocks of Electrochemical Cells
Electrochemical cells consist of two half-cells, each comprising an electrode immersed in a solution containing ions of the same element. The electrode serves as a conduit for electrons to flow in or out of the solution. The standard hydrogen electrode (SHE) is the reference point against which the reduction potentials of other half-cells are compared.
Each half-cell possesses a standard reduction potential, which represents the tendency of the ions in the solution to gain electrons and undergo reduction. This value is tabulated in standard reduction potential tables, providing a convenient reference for various electrode reactions.
Nernst Equation: Unveiling the Effects of Concentration and Temperature
The Nernst equation allows us to calculate the reduction potential of a half-cell under non-standard conditions, such as when the concentration of ions in the solution deviates from the standard value or when the temperature changes. This equation takes into account the influence of these factors on the reduction potential, enabling us to predict the behavior of half-cells in various scenarios.
Reduction Potential and Standard Reduction Potential
The reduction potential of a half-cell is the measure of its tendency to undergo reduction under specific conditions. It is directly related to the EMF of the electrochemical cell. The standard reduction potential is a special case of the reduction potential, measured when the concentrations of all reactants and products are at 1 M and the temperature is 298 K. Using standard reduction potentials from tables, we can easily calculate the EMF of an electrochemical cell.
Standard Cell Potential: The Driving Force Behind Electrochemical Reactions
The standard cell potential is the EMF of an electrochemical cell operating under standard conditions. It predicts the spontaneity of the cell reaction: a positive standard cell potential signifies a spontaneous reaction, while a negative value indicates a non-spontaneous reaction. Understanding the standard cell potential is crucial for designing and optimizing electrochemical processes.
Voltaic and Electrolytic Cells: Contrasting Modes of Operation
Electrochemical cells can be categorized into two types: voltaic cells and electrolytic cells. Voltaic cells generate electricity through spontaneous redox reactions, while electrolytic cells utilize an external power source to drive non-spontaneous reactions. The EMF of voltaic cells is a measure of the electrical energy produced, whereas the applied potential in electrolytic cells overcomes the resistance to the non-spontaneous reaction.
Calculating E° Cell: Unraveling the Power of Electrochemical Reactions
In the realm of chemistry, electrochemical cells play a pivotal role in converting chemical energy into electrical energy and vice versa. Electromotive force (EMF), also known as cell potential, is the driving force behind these transformations.
Half-Cells: The Building Blocks of Electrochemical Reactions
Electrochemical cells consist of two half-cells, each containing a metal electrode immersed in an electrolyte solution. Half-cells are where the magic happens—the oxidation and reduction reactions that generate or consume electrical energy.
The standard reduction potential of a half-cell, denoted as E°, measures its tendency to undergo reduction. It’s a key parameter that helps us determine the direction and extent of electrochemical reactions.
The Standard Hydrogen Electrode (SHE): A Benchmark for Reduction
The standard hydrogen electrode (SHE) serves as a reference point for measuring reduction potentials. Its E° is arbitrarily set to zero volts. By comparing the reduction potentials of other half-cells to the SHE, we can gain insights into their reactivity.
The Nernst Equation: Unlocking the Effects of Concentration and Temperature
The Nernst equation allows us to calculate the reduction potential of a half-cell under non-standard conditions, considering factors such as concentration and temperature. This equation is a powerful tool for predicting the behavior of electrochemical cells in real-world applications.
Reduction Potential and Standard Reduction Potential: Understanding the Driving Force
The reduction potential of a half-cell, denoted as E, is a measure of its ability to undergo reduction in a specific electrolyte solution. It’s related to the E° by the Nernst equation.
The standard reduction potential is the reduction potential of a half-cell under standard conditions (1 M concentration, 298 K). It’s a valuable reference value that enables us to predict the relative reactivity of half-cells and the overall cell potential.
Standard Cell Potential: The Sum of the Parts
The standard cell potential (E°cell) is the EMF of a cell under standard conditions. It represents the maximum electrical potential that the cell can generate. To calculate E°cell, we simply add the E° values of the two half-cells that make up the cell.
Voltaic and Electrolytic Cells: Two Sides of the Same Coin
Electrochemical cells can be divided into two main types: voltaic cells and electrolytic cells. Voltaic cells generate electricity from spontaneous chemical reactions, while electrolytic cells require an external electrical potential to drive non-spontaneous reactions.
The EMF of a voltaic cell is positive, indicating that the reaction is spontaneous. Conversely, the EMF of an electrolytic cell is negative, indicating that the reaction is non-spontaneous.
Understanding how to calculate EMF is crucial for comprehending and predicting the behavior of electrochemical cells. The standard reduction potential table and the Nernst equation are indispensable tools that allow us to explore the complex world of electrochemical reactions. By harnessing the power of EMF, we can design and optimize electrochemical devices for a wide range of applications, from batteries and fuel cells to sensors and electroplating.
Calculating E° Cell: A Comprehensive Guide to Understanding Electrochemical Processes
In the realm of electrochemistry, we encounter electrochemical cells, devices that harness the power of chemical reactions to produce electricity or vice versa. At the heart of these cells lies a crucial concept: electromotive force (EMF), which quantifies the electrical potential difference between two electrodes. To comprehend the intricate workings of electrochemical cells, we must delve into the calculation of EMF using the equation for E° cell.
Half-Cells and Standard Reduction Potentials
Electrochemical cells comprise two half-cells: one where oxidation occurs and the other where reduction takes place. Each half-cell has a unique reduction potential, a measure of its tendency to undergo reduction. The standard reduction potential of a half-cell is defined as its reduction potential when measured against the standard hydrogen electrode (SHE), a reference electrode with an assigned reduction potential of 0 V.
The Nernst Equation: Unveiling Non-Standard Conditions
Under non-standard conditions, such as varying concentrations and temperatures, the reduction potential of a half-cell deviates from its standard value. The Nernst equation provides a powerful tool to calculate this non-standard reduction potential:
E = E° - (RT/nF) * ln(Q)
Where:
- E is the non-standard reduction potential
- E° is the standard reduction potential
- R is the ideal gas constant, 8.314 J/mol·K
- T is the temperature in Kelvin
- n is the number of moles of electrons transferred in the half-reaction
- F is the Faraday constant, 96485 C/mol
- Q is the reaction quotient for the half-reaction
Standard Cell Potential: The Driving Force
The standard cell potential (E° cell) measures the electrical potential difference between the two half-cells under standard conditions. This value represents the maximum potential difference that can be achieved by the cell. The equation for calculating E° cell is:
E° cell = E° (cathode) - E° (anode)
Where:
- E° (cathode) is the standard reduction potential of the half-cell undergoing reduction
- E° (anode) is the standard reduction potential of the half-cell undergoing oxidation
Voltaic and Electrolytic Cells: A Tale of Two Processes
Electrochemical cells can be classified into two categories based on their operation:
- _Voltaic cells generate electricity from spontaneous chemical reactions. E° cell is positive for voltaic cells, indicating a spontaneous flow of electrons.
- _Electrolytic cells use an external electrical source to drive non-spontaneous chemical reactions. E° cell is negative for electrolytic cells, requiring an applied potential to overcome the nonspontaneous nature of the reaction.
Unveiling the Secrets of Electrochemical Cells: Calculating E° Cell
In the realm of electrochemistry, where electrons dance and energy flows, the concept of electromotive force (EMF) reigns supreme. It’s the driving force behind electrochemical cells, devices that harness the power of chemical reactions to generate electricity or facilitate chemical transformations.
But how do we determine the EMF of an electrochemical cell? That’s where the equation E° cell = E° red (cathode) – E° red (anode) comes into play. It’s a formula that unveils the relationship between the reduction potentials of the half-cells that make up the electrochemical cell.
The Symphony of Half-Cells
An electrochemical cell comprises two half-cells, each consisting of a metal electrode immersed in a solution of its metal ions. One half-cell is the cathode, where reduction occurs (electrons are gained), while the other is the anode, where oxidation occurs (electrons are lost).
Standard Reduction Potential: The Yardstick of Half-Cells
The standard reduction potential (E° red) of a half-cell is a measure of its tendency to undergo reduction. It’s assigned a value relative to the standard hydrogen electrode (SHE), which has an E° red of 0.00 V. The more positive the E° red, the greater the half-cell’s tendency to gain electrons and undergo reduction.
Putting it All Together: Calculating E° Cell
The EMF of an electrochemical cell is essentially the difference in reduction potentials between the cathode and anode half-cells. If the E° red (cathode) > E° red (anode), the reaction will be spontaneous and the cell will operate as a voltaic cell, generating electricity. Conversely, if E° red (cathode) < E° red (anode), an external potential is required to drive the reaction, making the cell an electrolytic cell.
A Deeper Dive: voltaic and Electrolytic Cells
-
Voltaic Cells:: In these cells, the chemical energy of the spontaneous reaction is converted into electrical energy. The EMF of the cell represents the maximum potential difference that can be achieved.
-
Electrolytic Cells:: Here, an external potential is applied to force a non-spontaneous reaction to occur. The applied potential must be greater than the EMF of the cell to overcome the reaction’s activation energy.
Calculating EMF is a fundamental skill in electrochemistry. It allows us to predict the spontaneity of reactions, design electrochemical cells, and understand the underlying thermodynamics of these processes. Armed with this knowledge, we can harness the power of electrochemistry for a wide range of applications, from powering devices to driving chemical reactions.
Calculating E° Cell: Unlocking the Power of Electrochemistry
Imagine a battery, the lifeblood of our electronic devices. Behind its humble exterior lies a fascinating world of electrochemistry, governed by the concept of electromotive force (EMF). EMF is the driving force that enables electrochemical cells to convert chemical energy into electrical energy, powering our gadgets and countless other applications. To understand this hidden power, we embark on a journey of calculating E° cell, the standard cell potential.
Half-Cells: The Building Blocks of Electrochemical Cells:
An electrochemical cell consists of two half-cells, each containing a different electrode immersed in a solution. One half-cell is where oxidation occurs, releasing electrons, while the other is where reduction takes place, consuming electrons. The key to understanding electrochemical cells is the concept of the standard reduction potential, a measure of how easily a substance can undergo reduction.
The Standard Hydrogen Electrode: The Benchmark for Reduction:
The standard reduction potential is measured against a reference electrode known as the standard hydrogen electrode (SHE). By assigning a reduction potential of zero to SHE, we have a universal benchmark against which all other reduction potentials are compared. This allows us to quantify and predict the behavior of electrochemical cells.
Obtaining Standard Reduction Potentials: A Table of Secrets:
Standard reduction potentials are tabulated in reference tables, providing a treasure trove of information about the relative ease of reduction for various substances. These tables are like maps of the electrochemical landscape, guiding us in predicting the direction of spontaneous reactions and calculating E° cell.
Standard Cell Potential: The Driving Force of Electrochemistry:
The standard cell potential, E° cell, represents the maximum EMF that can be generated by an electrochemical cell under standard conditions. It is calculated as the difference between the standard reduction potentials of the two half-cells involved. A positive E° cell indicates a spontaneous reaction, while a negative E° cell indicates a non-spontaneous reaction.
Voltaic and Electrolytic Cells: Two Sides of the Electrochemical Coin:
Electrochemical cells can be categorized into two types: voltaic and electrolytic. Voltaic cells, like batteries, generate electricity from spontaneous chemical reactions, while electrolytic cells use applied electrical energy to drive non-spontaneous chemical reactions. Understanding E° cell is crucial for predicting the behavior and efficiency of these cells.
Calculating E° cell is a fundamental skill for comprehending electrochemical processes. By mastering this concept, we gain insight into the inner workings of batteries, fuel cells, and countless other applications that harness the power of electrochemistry. The tables of standard reduction potentials and the Nernst equation are our guiding lights in this fascinating realm, where chemical energy and electrical energy dance in perfect harmony.
Calculating E° Cell: Unveiling the Electrical Power of Electrochemical Processes
In the realm of electrochemistry, a crucial concept emerges: electromotive force (EMF), the driving force behind electrochemical reactions. EMF holds significant importance in determining the spontaneity and energy conversion capabilities of electrochemical cells. To accurately predict EMF, we delve into the intriguing world of standard cell potential.
Standard Cell Potential: Uncovering the Intrinsic Energy of Electrochemical Cells
Standard cell potential, denoted by E° cell, quantifies the electrical potential difference between two electrodes in an electrochemical cell under standard conditions (1 M concentration of reactants, temperature of 298 K, and pressure of 1 atm). It provides valuable insights into the spontaneity and extent of electrochemical reactions.
The standard cell potential can be calculated using the following equation:
E° cell = E° red (cathode) - E° red (anode)
where:
- E° red (cathode) is the standard reduction potential of the substance undergoing reduction (gaining electrons)
- E° red (anode) is the standard reduction potential of the substance undergoing oxidation (losing electrons)
Standard Reduction Potentials: A Reference Point for Electrochemical Reactions
Standard reduction potentials, often tabulated in textbooks, offer a convenient way to determine the relative reactivity of various substances in electrochemical reactions. The more positive the standard reduction potential, the greater the tendency of the substance to undergo reduction. Values are typically reported relative to the standard hydrogen electrode (SHE), which has a standard reduction potential of 0.00 V.
Calculating E° Cell: A Journey of Chemical and Electrical Understanding
By combining the concept of standard reduction potentials with the equation for calculating E° cell, we gain the ability to predict the outcome of electrochemical reactions. A positive E° cell value indicates a spontaneous reaction, while a negative value suggests a non-spontaneous reaction. Furthermore, the magnitude of E° cell provides information about the extent of the reaction’s spontaneity and the energy conversion efficiency.
Calculating E° cell is a fundamental aspect of electrochemistry, enabling us to understand the behavior of electrochemical systems, predict reaction spontaneity, and harness the power of electrical energy conversion. Armed with this knowledge, scientists and engineers can push the boundaries of electrochemical applications, including the development of efficient batteries, fuel cells, and other energy-related technologies.
Calculating E° Cell: A Guide to Understanding Electrochemical Processes
Electrochemical cells are fascinating devices that harness the power of chemical reactions to generate electricity or drive non-spontaneous reactions. Understanding the intricacies of these cells requires a thorough grasp of electromotive force (EMF), which we will delve into in this blog post.
Standard Cell Potential: The Key to Predicting Cell Behavior
The standard cell potential, denoted by E° cell, is a crucial parameter that predicts the spontaneity of electrochemical reactions. It is calculated using the equation:
E° cell = E° (reduction) – E° (oxidation)
where E° (reduction) and E° (oxidation) represent the standard reduction potentials of the reduction and oxidation half-cells, respectively. These values can be obtained from tables of standard reduction potentials.
Standard Reduction Potentials: The Yardstick for Half-Cells
In electrochemical cells, reactions occur in two parts: reduction and oxidation. Standard reduction potentials measure the tendency of a half-cell to undergo reduction. The more positive the standard reduction potential, the greater the tendency of the half-cell to undergo reduction.
The standard hydrogen electrode (SHE) serves as the reference against which all other reduction potentials are compared. By convention, the SHE has a standard reduction potential of 0.00 V.
Nernst Equation: Predicting Cell Potential under Non-Standard Conditions
Under non-standard conditions, such as variations in temperature or concentration, the reduction potential of a half-cell can be calculated using the Nernst equation:
E° cell = E° (reduction) – (RT/nF) * ln(Q)
where R is the gas constant, T is the temperature in Kelvin, n is the number of electrons transferred in the reaction, F is Faraday’s constant, and Q is the reaction quotient.
Calculating EMF is essential for understanding the behavior of electrochemical cells. Standard reduction potentials and the Nernst equation provide valuable tools for predicting cell potential and the spontaneity of electrochemical reactions. This knowledge is indispensable for various applications, including the development of batteries, fuel cells, and other electrochemical devices.
**Calculating E° Cell: A Step-by-Step Guide**
In the realm of electrochemistry, the electromotive force (EMF) plays a pivotal role, governing the flow of electrons in electrochemical cells. Whether it’s the power source in a battery or the driving force behind electrodeposition, understanding EMF is essential.
At the heart of electrochemical cells lies the enigmatic half-cell. Half-cells are electrochemical vessels where one of two complementary reactions occurs: oxidation or reduction. Think of them as the yin and yang of chemical reactions, each carrying out its designated task.
To quantify the individual contributions of half-cells, scientists have devised the standard reduction potential. This value represents the potential difference between a given half-cell and the standard hydrogen electrode (SHE), an unwavering reference point with a reduction potential of 0.00 volts.
Just as explorers use a map and compass to navigate the wilderness, electrochemists rely on the Nernst equation to calculate the reduction potential of half-cells under various conditions. This equation factors in temperature, concentration, and other variables, allowing us to adapt our predictions to real-world scenarios.
Reduction Potential and Standard Reduction Potential are two closely related concepts. Reduction potential refers to the potential difference of a specific half-cell, while standard reduction potential is its reference value, measured against the SHE. These values provide valuable insights into the relative strength of reducing and oxidizing agents.
All roads lead to Rome, and in electrochemistry, all half-cell potentials lead to the standard cell potential (E° cell). This crucial value represents the overall EMF of an electrochemical cell under standard conditions and is calculated as the difference in standard reduction potentials of the constituent half-cells.
In the electrochemical realm, two distinct types of cells emerge: voltaic and electrolytic. Voltaic cells, the natural powerhouses, harness spontaneous reactions to generate electricity. Electrolytic cells, on the other hand, require an external electrical force to drive non-spontaneous reactions.
Voltaic cells are the star performers of elektrochemistry, generating electricity through spontaneous reactions. Think of them as tiny power plants, converting chemical energy into electrical energy. In contrast, electrolytic cells rely on an external electrical force to push non-spontaneous reactions in the desired direction. These cells are used in various applications, including electroplating and the production of hydrogen fuel.
In conclusion, calculating E° Cell is a fundamental skill in electrochemistry, providing access to a wealth of insights into electrochemical processes. From understanding the spontaneity of reactions to designing efficient batteries, this knowledge empowers scientists and engineers in countless fields.
Calculating Electromotive Force (EMF) in Electrochemical Cells: A Comprehensive Guide
Half-Cells and Standard Reduction Potential
Electrochemical cells consist of two half-cells, each with a metal electrode immersed in a solution containing its ions. The standard reduction potential of a half-cell is a measure of its tendency to gain electrons, with more positive values indicating a greater tendency. The standard hydrogen electrode (SHE) serves as the reference half-cell, with a standard reduction potential of 0.00 V.
Nernst Equation
The Nernst equation allows us to calculate the reduction potential of a half-cell under non-standard conditions. It accounts for changes in concentration, temperature, and pressure.
Standard Cell Potential
Standard cell potential (E° cell) is the EMF of an electrochemical cell when all reactants and products are at standard state conditions (1 M concentration, 298 K, 1 atm pressure). It can be calculated using the equation:
E° cell = E° reduction (cathode) - E° reduction (anode)
where:
- E° reduction (cathode) is the standard reduction potential of the cathode half-cell
- E° reduction (anode) is the standard reduction potential of the anode half-cell
Voltaic and Electrolytic Cells
Voltaic cells are spontaneous reactions that generate electricity. The EMF of a voltaic cell is positive and drives the flow of electrons from the anode to the cathode. Electrolytic cells, on the other hand, are non-spontaneous reactions that require an applied potential to drive the flow of electrons in the opposite direction, from the cathode to the anode.
Calculating EMF is crucial for understanding electrochemical processes. Standard reduction potential tables and the Nernst equation provide valuable tools for determining the EMF of half-cells and electrochemical cells. These principles have wide applications in fields such as battery design, corrosion prevention, and electrochemical sensing.
Calculating E° Cell: Unlocking the Secrets of Electrochemical Reactions
Embark on a captivating journey into the realm of electrochemistry, where we unravel the mysteries of electromotive force (EMF), a fundamental concept that governs electrochemical processes. In this blog post, we’ll equip you with the tools to calculate E° cell, the driving force behind electrochemical reactions.
Understanding the Significance of EMF
Imagine a bustling city where electrons, like tiny commuters, flow freely between different regions. The electromotive force (EMF) is the force that drives this electron flow, akin to voltage in an electrical circuit. In electrochemical cells, EMF determines the spontaneity and direction of chemical reactions. By calculating EMF, we can predict the behavior and efficiency of these reactions.
Deciphering Half-Cells
Electrochemical cells are composed of two half-cells, each containing a different electrode submerged in an electrolyte solution. One electrode undergoes oxidation, losing electrons, while the other undergoes reduction, gaining electrons. The standard reduction potential of each half-cell, a measure of its tendency to undergo reduction, holds the key to understanding cell behavior.
Exploring the Nernst Equation
The Nernst equation unveils the enigmatic relationship between reduction potential and non-standard conditions such as concentration and temperature. It allows us to calculate the reduction potential of half-cells under varying circumstances, providing valuable insights into cell operation.
Unveiling Reduction and Standard Reduction Potentials
The reduction potential of a half-cell is a crucial factor in determining EMF. Standard reduction potential, a tabulated value, represents the reduction potential of a half-cell under standard conditions. By comparing standard reduction potentials, we can determine the spontaneity of a reaction and its potential for electron flow.
Calculating Standard Cell Potential
The standard cell potential (E° cell) is the difference in standard reduction potentials between the two half-cells involved in a reaction. E° cell provides invaluable information about the favorability and efficiency of the reaction. A positive E° cell indicates a spontaneous reaction, while a negative value signifies a non-spontaneous reaction.
Distinguishing Voltaic and Electrolytic Cells
Electrochemical cells come in two distinct flavors: voltaic and electrolytic. Voltaic cells harness spontaneous reactions to generate electrical energy, akin to batteries. In contrast, electrolytic cells require an external electrical source to drive non-spontaneous reactions, such as electrolysis processes.
The Importance of Understanding EMF
Calculating EMF empowers us to comprehend the intricate workings of electrochemical processes. It’s an essential tool for predicting cell behavior, designing efficient batteries, developing new electrochemical technologies, and unraveling the mysteries of electrochemistry in various fields such as energy storage, corrosion control, and chemical synthesis.
Calculating E° Cell: A Comprehensive Guide for Understanding Electrochemical Processes
In the realm of electrochemistry, electromotive force (EMF) is a crucial concept that governs the behavior of electrochemical cells. EMF, also known as cell potential, measures the tendency of a chemical reaction to proceed spontaneously. In this blog post, we will delve into the intricacies of calculating E° cell, the standard cell potential, which provides valuable insights into electrochemical processes.
Half-Cells and Standard Reduction Potentials:
Electrochemical cells consist of two half-cells, each with its own electrode and electrolyte solution. The standard reduction potential of a half-cell is a measure of its tendency to undergo reduction under standard conditions. The standard reduction potential of the standard hydrogen electrode (SHE) is assigned a value of 0.00 V.
Nernst Equation and Reduction Potentials:
In non-standard conditions, the reduction potential of a half-cell can be calculated using the Nernst equation. This equation accounts for factors such as temperature, concentration, and pressure. By understanding the Nernst equation, we can determine the reduction potential of half-cells under varying circumstances.
Standard Cell Potential and Voltaic Cells:
Standard cell potential (E° cell) is the EMF of a cell under standard conditions. It can be calculated by subtracting the reduction potential of the anode from the reduction potential of the cathode. In voltaic cells, the spontaneous electrochemical reaction generates an electrical current. The EMF of voltaic cells is directly proportional to the cell potential.
Electrolytic Cells and Applied Potential:
Electrolytic cells, on the other hand, require an applied potential to drive a non-spontaneous reaction. The applied potential is greater than the opposing EMF of the cell. This allows for the electrolysis of various compounds, a process that has numerous practical applications.
Applications of Standard Reduction Potentials and the Nernst Equation:
Standard reduction potential tables and the Nernst equation are invaluable tools in various applications, including:
- Predicting the feasibility of reactions: By comparing the reduction potentials of the reactants and products, we can determine if a reaction is spontaneous or non-spontaneous.
- Designing electrochemical cells: Understanding E° cell and the Nernst equation is essential for optimizing the performance of electrochemical cells for specific applications.
- Developing electroanalytical methods: Electrochemical techniques, such as voltametry and amperometry, rely on measuring the EMF of electrochemical cells to analyze chemical species.
- Corrosion protection: Standard reduction potentials can provide insights into the corrosion susceptibility of metals and alloys.
Calculating E° cell is a fundamental aspect of electrochemistry, providing a quantitative measure of the spontaneity and direction of electrochemical reactions. Standard reduction potential tables and the Nernst equation are indispensable tools for understanding and manipulating electrochemical processes in various applications. By mastering these concepts, we gain a deeper understanding of the fascinating world of electrochemistry.