Bromine: Unraveling The Atomic Configuration And Electron Distribution
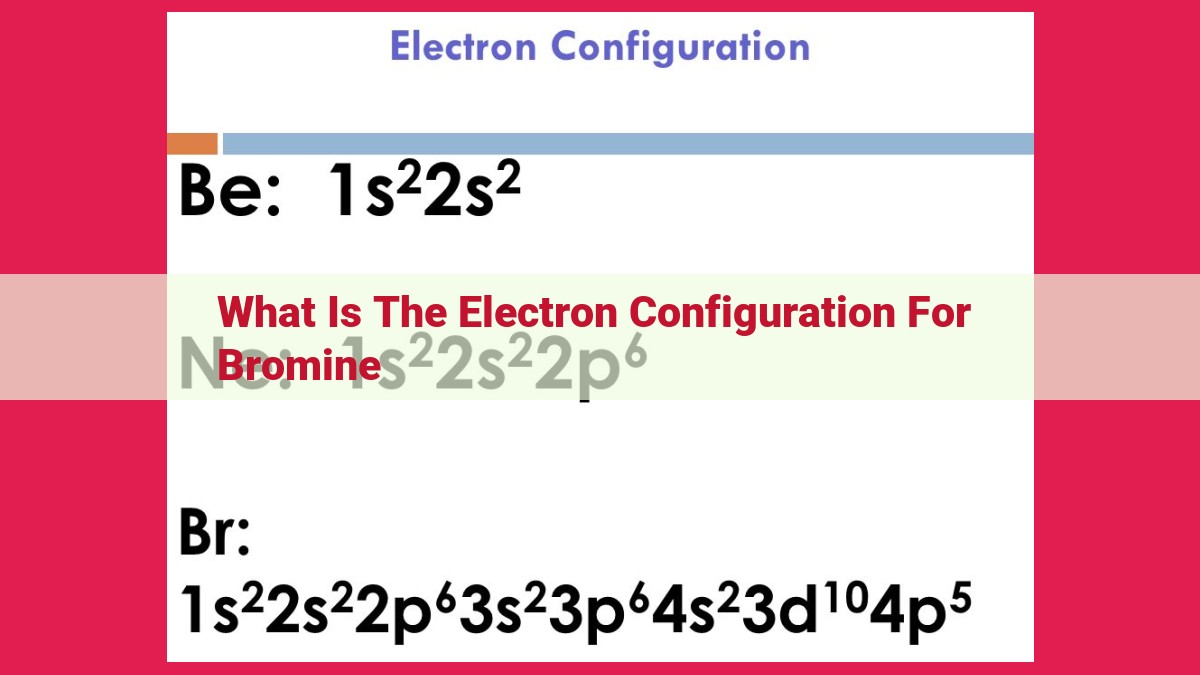
Bromine, with an atomic number of 35, has an electron configuration of [Ar] 3d¹⁰ 4s² 4p⁵. This means that it has 35 electrons distributed in its orbitals as follows: 18 in the argon-like core configuration, 10 in the 3d subshell, 2 in the 4s subshell, and 5 in the 4p subshell.
Atomic Number: The Unique Key to the Elements
In the vast tapestry of the universe, each element stands as a distinct entity, bearing its own identity. This identity is defined by its atomic number, a fundamental property that plays a pivotal role in the element’s characteristics and behavior. It is the unique number of protons residing in the nucleus of an atom.
The atomic number not only serves as a fingerprint for each element but also holds profound implications for its atomic structure and chemical properties. It establishes a direct relationship with the number of electrons surrounding the nucleus, as well as the number of neutrons that complete the atomic core. In essence, the atomic number provides a comprehensive blueprint for understanding the building blocks of any element.
By unveiling the atomic number, scientists have gained invaluable insights into the fundamental nature of matter and the intricate relationships that govern the chemical world. It has become an indispensable tool for predicting the behavior of elements, designing new materials, and unraveling the mysteries of the cosmos.
Exploring Electron Configuration: Mapping the Abode of Electrons
Electrons, those tiny and elusive particles that dance around atoms, hold the key to understanding the intricate tapestry of our world. Delving into the realm of electron configuration is a journey that illuminates the mysteries of atomic structure and chemical behavior.
Unveiling the Electron Distribution
Visualizing electron distribution is no easy feat, but orbital notation comes to our rescue. This ingenious tool allows us to depict the arrangement of electrons within an atom’s orbitals, the energy levels where they reside. Just like houses have rooms, orbitals represent specific energy zones, and electrons occupy these spaces like tiny tenants.
The Rules of the Electron Neighborhood
Within this atomic neighborhood, certain principles govern how electrons behave. The Pauli exclusion principle declares that no two electrons can share the same exact spot, ensuring a unique identity for each electron. Hund’s rule, on the other hand, dictates that electrons favor having their own space by occupying different orbitals with parallel spins. And the Aufbau principle ensures order by guiding electrons to fill orbitals in a stepwise manner, starting from the lowest energy level.
The Noble Gas Sanctuary
In the electron configuration world, there’s a coveted state of stability known as the noble gas configuration. These elements, like the enigmatic helium or the aloof argon, have completely filled outer orbitals. This electron arrangement grants them an air of contentment, rendering them chemically inert and unwilling to participate in interactions with their neighbors.
Additional Tips for SEO:
- Use relevant keywords: Include terms like “electron configuration,” “orbital notation,” and “Pauli exclusion principle” throughout your content.
- Optimize headings: Structure your headings using H2 and H3 tags for subheadings to improve readability and crawlability.
- Use alt tags for images: Add descriptive alt text to any images you include to improve accessibility and provide context to search engines.
- Link to reputable sources: Cite scientific articles or authoritative websites to establish credibility and provide further information for readers.
Valence Electrons: The Gatekeepers of Chemical Reactivity
The world of chemistry revolves around the intricate dance of electrons, those tiny particles that determine the properties and behaviors of every element. Among these electrons, a special group known as valence electrons holds the key to a substance’s chemical reactivity.
Imagine a group of children playing in a playground, each with their own unique set of toys. Valence electrons are like these children, eager to interact and play with other electrons. They occupy the outermost energy level of an atom, making them the first point of contact for other atoms.
The number of valence electrons an atom possesses is critically important. It governs the element’s ability to form chemical bonds with other elements, determining its chemical properties. For instance, elements with a high number of valence electrons, such as chlorine and oxygen, are highly reactive and readily form bonds with other elements. In contrast, elements with a low number of valence electrons, such as helium and neon, are less reactive and tend to form bonds only under specific conditions.
Moreover, valence electrons follow certain periodic trends that play a crucial role in understanding their chemical behavior. As we move down a group (vertical column) of the periodic table, the number of valence electrons increases. This trend is responsible for the increased reactivity of elements within a group. Moving across a period (horizontal row), the number of valence electrons increases from left to right. This change in valence electrons influences the chemical properties of elements, leading to gradual variations in their reactivity.
The octet rule is a fundamental concept in chemistry that governs the behavior of valence electrons. This rule states that atoms tend to be most stable when they have eight valence electrons, a so-called octet. Elements that follow the octet rule are noble gases, which are highly stable and unreactive. Other elements strive to achieve an octet of valence electrons through chemical bonding, which involves sharing or transferring electrons with other atoms.
Lewis structures are powerful tools that allow us to visualize the arrangement of valence electrons in molecules. These structures represent molecules as a collection of symbols for atoms connected by lines or dots, which represent shared electron pairs. Lewis structures are invaluable in predicting the chemical behavior of molecules by helping us understand how valence electrons interact.
By unraveling the secrets of valence electrons, we gain a deeper understanding of the fundamental forces that drive chemical reactions. These tiny particles play a pivotal role in determining the properties of substances, shaping the world around us in countless ways.
Noble Gas Configuration: The Pinnacle of Electron Stability
In the vast world of elements, there exists a select group of chemical nobility—the inert gases or noble gases. Helium, neon, argon, krypton, xenon, and radon: these elements possess a remarkable atomic characteristic that sets them apart from the rest—a stable electron configuration.
The electron configuration of an atom refers to the arrangement of its electrons in energy levels or orbitals. For the noble gases, this arrangement holds a profound significance, as it grants them unparalleled stability.
The Group 18 elements—the noble gases—all share a unique property: their outermost energy level is completely filled. This octet rule configuration, with eight electrons in the outermost energy level, creates an exceptionally stable state, making these elements unreactive.
In contrast, other elements tend to form chemical bonds with other atoms in an attempt to achieve a stable octet configuration. However, the noble gases have already reached this electron arrangement, rendering them inert in most chemical reactions.
The noble gases’ stability allows them to play crucial roles in various applications. Helium, for instance, is used in balloons and airships due to its low density and non-reactivity. Neon finds its niche in lighting fixtures, emitting a soft, warm glow. Argon is employed in welding and incandescent light bulbs, creating a protective atmosphere that prevents oxidation.
Understanding the noble gas configuration provides a cornerstone for comprehending atomic structure and chemical behavior. The stability of these elements unveils the significance of electron arrangement and the driving force behind chemical reactions.
Orbital Notation: Deciphering the Electron Dance
In the realm of elements, electrons occupy a crucial role, determining the chemistry and properties of the matter around us. To unravel the intricate world of electrons, we turn to orbital notation, a visual guide that unveils the distribution patterns of these minuscule particles.
A Quantum Leap into Orbital Mechanics
Delving into the fascinating world of quantum mechanics, we encounter the concept of atomic orbitals, ethereal regions where electrons reside. These orbitals take on various shapes, akin to clouds surrounding an atomic nucleus. Each orbital can accommodate a maximum of two electrons, with their spins aligned either parallel or antiparallel.
Electron Spin: A Tale of Two Twists
Electrons possess an intrinsic property known as spin, analogous to tiny spinning tops. This spin can be either “up” or “down,” a crucial factor in determining the overall stability of an atom. Hund’s rule, a fundamental principle, dictates that electrons within the same orbital will align their spins in a parallel fashion, maximizing their stability.
Orbital Notation: Mapping the Electron Metropolis
Orbital notation provides a graphical representation of electron distribution using a set of symbols:
- s orbitals: Spherical in shape, resembling halos around the nucleus.
- p orbitals: Resembling dumbbells, oriented along the x, y, and z axes.
- d orbitals: More complex shapes, often resembling four-leaf clovers or bow ties.
- f orbitals: Even more intricate shapes, found in heavier elements.
Aufbau Principle: Electron Placement from the Ground Up
As electrons populate orbitals, they follow a specific order governed by the Aufbau principle. This principle states that electrons will fill the lowest energy orbitals first, progressing to higher energy levels as needed.
Implications for Atomic Structure and Properties
Orbital notation reveals the profound influence of electron distribution on atomic structure. The number of unpaired electrons determines an atom’s magnetic properties, as unpaired electrons impart a magnetic field to the atom. Moreover, orbital configurations dictate an atom’s chemical reactivity and bonding preferences.
Electron Configuration Notation: A Universal Language for Electrons
- Using orbital notation to represent electron distribution
- s, p, d, and f orbitals and their shapes
Electron Configuration Notation: A Universal Language for Electrons
Imagine the atom as a miniature solar system, with electrons orbiting the nucleus like planets. To describe these orbits, scientists have developed a special language called electron configuration notation. This notation provides a visual representation of where electrons are located within an atom.
Electron configuration notation uses orbital notation, a system that represents the precise distribution of electrons in each orbital. Orbitals are the specific regions within an atom where electrons are most likely to be found. Each orbital has a unique shape and energy level.
The four main types of orbitals are denoted by the letters s, p, d, and f. s orbitals are spherical, p orbitals are dumbbell-shaped, d orbitals have complex shapes with five lobes, and f orbitals have even more complex shapes. Each orbital can hold a maximum of two electrons.
Electron configuration notation follows a specific set of rules:
- Electrons fill orbitals from lowest to highest energy level.
- Within each energy level, orbitals are filled in sublevels.
- Each sublevel can hold a maximum of six electrons.
- Electrons are arranged so that the total spin* alignment is maximized (Hund’s rule).
By using electron configuration notation, scientists can represent the electron configuration of any element. This information is invaluable for understanding an element’s chemical behavior, as it determines its ability to form chemical bonds with other elements.
Aufbau Principle: Guiding the Electron Filling Order
- Rules governing how electrons fill orbitals
- Implications for atomic structure and properties
Aufbau Principle: Building the Foundation of Atomic Structure
In the realm of atomic physics, understanding the arrangement of electrons within atoms is crucial. The Aufbau principle is a fundamental concept that guides this electron distribution, providing a roadmap for how electrons fill orbitals.
Orbitals: The Electron’s Abode
Orbitals are three-dimensional regions within an atom where electrons are most likely to be found. Each orbital has a specific shape and energy level, and Aufbau principle dictates the order in which electrons occupy these orbitals.
Rules of the Aufbau Principle
The Aufbau principle is governed by two key rules:
-
Lowest Energy First: Electrons first fill the lowest energy orbitals available. Orbitals closer to the nucleus, with a lower principal quantum number n, have lower energy.
-
Hund’s Rule: When there are multiple orbitals with the same energy level, electrons initially fill them with parallel spins. This maximizes the total electron spin, contributing to the atom’s stability.
Implications for Atomic Structure
The electron distribution resulting from the Aufbau principle has profound implications for atomic structure:
-
Size and Shape of Atoms: The number and arrangement of electrons determine the size and shape of atoms. Atoms with more electrons are larger, while those with electrons in higher energy levels have more complex shapes.
-
Reactivity and Chemical Properties: Electrons in the outermost orbitals, known as valence electrons, play a crucial role in chemical bonding and reactivity. The Aufbau principle helps predict the chemical behavior of elements based on their electron configurations.
The Aufbau principle is a cornerstone of our understanding of atomic structure and behavior. By providing a framework for electron distribution, it enables us to unravel the mysteries of chemical bonding, reactivity, and the countless phenomena that shape our physical world.
Hund’s Rule: Maximizing Electron Stability
Introduction
The enigmatic world of quantum mechanics governs the behavior of electrons within atoms. Among the fascinating principles that shape their arrangement is Hund’s rule, a guiding force that maximizes electron stability. This rule dictates how electrons align their spins within orbitals, profoundly influencing an atom’s magnetic properties and overall stability.
Electron Spin and Stability
Like tiny magnets, electrons possess a property known as spin, which can be either “up” or “down”. Hund’s rule states that when multiple electrons occupy the same orbital (a region where electrons are most likely to be found), they will prefer to have their spins parallel to each other. This parallel alignment lowers the energy of the atom, making it more stable.
Implications for Paramagnetism
The presence of unpaired electrons, those with opposite spins, can give rise to a phenomenon called paramagnetism. Paramagnetism occurs when substances are attracted to magnetic fields due to the unpaired electrons’ magnetic moments. The greater the number of unpaired electrons, the stronger the paramagnetic behavior.
Hund’s Rule in Action
To illustrate Hund’s rule in practice, consider an atom with three electrons in the same orbital. According to the rule, these electrons will arrange themselves with parallel spins, resulting in two unpaired electrons. This high-spin configuration provides the atom with greater stability and paramagnetism.
Hund’s rule is a fundamental principle in atomic physics that governs the alignment of electron spins. It plays a crucial role in determining electron stability, magnetic properties, and overall atomic structure. By maximizing electron stability, Hund’s rule shapes the very nature of matter and the behavior of its building blocks.
Pauli Exclusion Principle: The Fundamental Law of Electron Behavior
In the realm of quantum mechanics, electrons dance within atoms, each occupying a unique abode defined by their spin orientation. This fundamental law of electron behavior, known as the Pauli Exclusion Principle, governs the very fabric of matter.
Imagine electrons as tiny magnets, each with a north pole and a south pole. Like two magnets of the same polarity, electrons cannot share the same quantum state. They must possess opposite spins, ensuring their unwavering individuality. This principle underpins the foundation of atomic structure, dictating how electrons arrange themselves within energy levels.
The Pauli Exclusion Principle not only dictates the behavior of individual electrons; it has profound implications for atomic and molecular structures. It explains why atoms have specific electron configurations, each with a unique set of energy levels and chemical properties. Moreover, it underpins the stability of molecules, preventing electrons from collapsing into the nucleus, forming a chaotic mess.
The Pauli Exclusion Principle stands as a testament to the remarkable precision of quantum mechanics, guiding the behavior of electrons and shaping the very nature of matter. It is a fundamental law of nature, an unbreakable rule that governs the subatomic world.