Boiling Point: A Comprehensive Guide To Understanding And Application
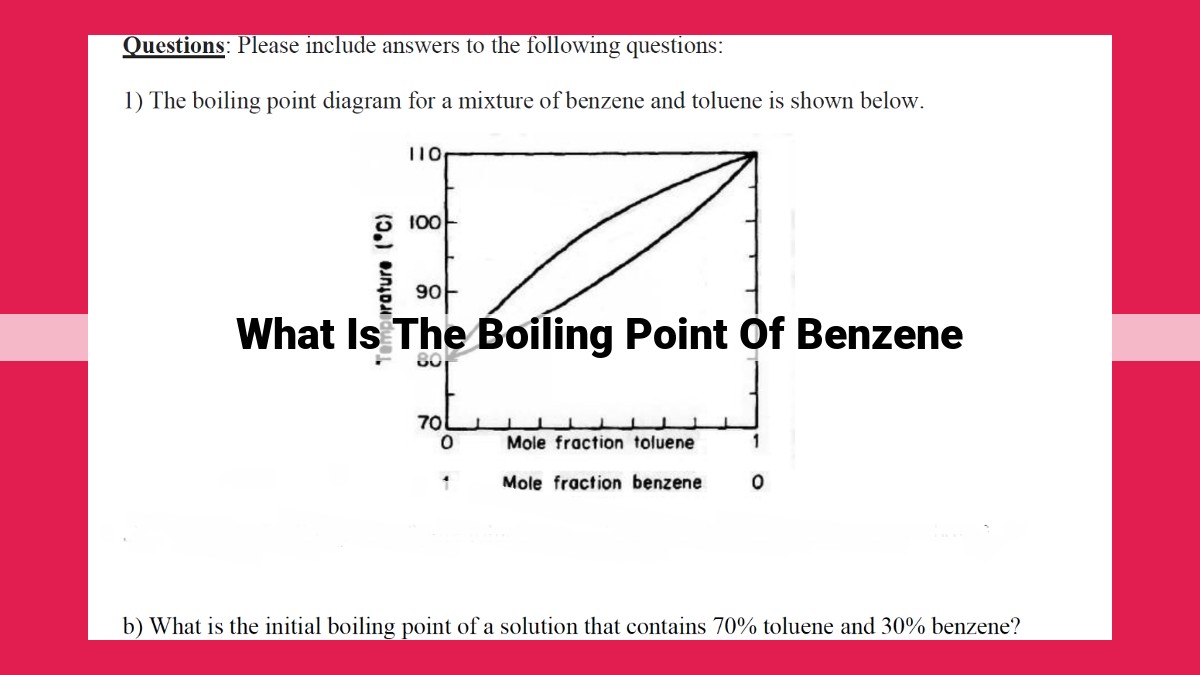
The normal boiling point of a liquid is the temperature at which its vapor pressure equals the atmospheric pressure. Intermolecular forces, such as van der Waals forces, hydrogen bonding, and dipole-dipole interactions, influence boiling point. Molecular weight plays a role, with heavier molecules typically having higher boiling points. Molecular structure, including symmetry and polarity, also affects boiling point. Atmospheric pressure affects boiling point, with higher pressures leading to higher boiling points. Vapor pressure and boiling point are linked through equilibrium. Temperature and boiling point have a direct relationship. For benzene, its boiling point is 80.1 °C (176.18 °F) at standard atmospheric pressure (101.325 kPa). Understanding boiling point is important in various fields, including chemistry, physics, and engineering.
Definition of Normal Boiling Point
- Explain the concept of normal boiling point and its significance.
The Enigmatic Dance of Molecules: Delving into the Intriguing World of Boiling Points
Boiling, a seemingly mundane phenomenon, conceals a captivating tale of molecular interactions and environmental influences. At its core lies the concept of normal boiling point, a pivotal juncture where a liquid transforms into a gas at atmospheric pressure. This metamorphosis marks a critical threshold in a substance’s journey, offering scientists a window into the intricate workings of matter.
Beyond its scientific significance, boiling point plays a pivotal role in countless everyday applications, shaping our lives in both overt and subtle ways. From the tantalizing aromas of simmering sauces to the efficient operation of steam turbines, understanding boiling points empowers us to harness the power of temperature and pressure to enhance our daily experiences.
The Invisible Symphony of Intermolecular Forces
The dance of molecules that culminates in boiling is governed by the interplay of intermolecular forces. These subtle yet powerful interactions determine the strength of the “bonds” holding liquid molecules together. Stronger intermolecular forces translate into a more tightly knit molecular collective, requiring greater energy (in the form of heat) to overcome and initiate boiling. Conversely, weaker intermolecular forces allow molecules to slip past each other more easily, resulting in lower boiling points.
The Molecular Weight Quandary: Size Does Matter
Molecular weight, a measure of the mass of a molecule, also exerts a profound influence on boiling points. Heavier molecules, laden with more atoms, possess greater inertia. This increased mass makes it more challenging to mobilize these molecules, leading to higher boiling points. Conversely, lighter molecules, with their nimble agility, can more readily break free from their liquid embrace, resulting in lower boiling points.
The Dance of Symmetry and Polarity: Molecular Structure Takes the Stage
The intricate tapestry of molecular structure adds an additional layer of complexity to the boiling point equation. Symmetrical molecules, with their uniform distribution of charge, experience weaker intermolecular forces. This molecular harmony translates into lower boiling points. Conversely, asymmetrical molecules, with their uneven charge distribution, encounter stronger intermolecular forces. These molecular asymmetries result in higher boiling points.
The Atmospheric Waltz: Pressure’s Grand Influence
Atmospheric pressure, the weight of the air above, plays a crucial role in the boiling point drama. At lower atmospheric pressures, such as those encountered at higher altitudes, molecules face less resistance to their transformation into gases. This reduced resistance leads to lower boiling points. Conversely, at higher atmospheric pressures, such as those encountered at sea level, molecules encounter greater resistance, resulting in higher boiling points.
The Delicate Interplay: Vapor Pressure and Boiling Point
Vapor pressure, the pressure exerted by molecules in the gas phase above a liquid, holds a mirror to the boiling point. As vapor pressure increases, the number of molecules escaping the liquid’s embrace intensifies. This increase in molecular mobility lowers the boiling point, as fewer molecules remain in the liquid state. Conversely, as vapor pressure decreases, more molecules remain captive in the liquid, raising the boiling point.
The Temperature Tango: A Direct Relationship
Temperature, the measure of molecular motion, dances in perfect harmony with boiling point. As temperature rises, molecular motion intensifies, leading to more frequent collisions and weaker intermolecular forces. This molecular symphony results in lower boiling points. Conversely, as temperature falls, molecular motion slows, strengthening intermolecular forces and raising boiling points.
Benzene’s Boiling Point: A Case Study
Benzene, an aromatic hydrocarbon, offers a revealing case study in the interplay of factors governing boiling point. With a relatively low molecular weight of 78.11 g/mol and a symmetrical molecular structure, benzene exhibits a boiling point of 80.1 °C (176.18 °F) at atmospheric pressure. This value reflects the delicate balance between its intermolecular forces, molecular weight, and molecular structure.
The Symphony’s Significance: Far-Reaching Applications
Understanding boiling points transcends academic curiosity, extending into a multitude of practical applications. From the design of chemical processes to the optimization of energy systems, boiling points guide scientists and engineers in harnessing the transformative power of temperature and pressure. In the culinary realm, boiling points dictate cooking times and ensure culinary masterpieces.
In the grand symphony of chemistry, boiling points resonate as critical notes, revealing the intricate interplay of molecular interactions and environmental influences. By unraveling the secrets of this molecular dance, we gain not only a deeper understanding of the world around us but also the tools to shape it to our advantage.
Influence of Intermolecular Forces on Boiling Point
In the tumultuous realm of matter, boiling point serves as a critical parameter that governs the transition from liquid to vapor. Intermolecular forces, the invisible hands that bind molecules together, play a pivotal role in shaping this defining characteristic.
Just as dancers entwined in an intricate waltz, molecules engage in a delicate dance, their movements dictated by the strength of their mutual attraction. Weak intermolecular forces, akin to a gentle breeze, allow molecules to slip past each other with relative ease. Strong intermolecular forces, on the other hand, resemble a sturdy embrace, locking molecules in place.
Consider the van der Waals forces that act between nonpolar molecules, such as helium and methane. These forces are like whispers between molecules, exerting a feeble influence on their separation. Consequently, substances like helium and methane possess low boiling points, as their molecules can break away from each other with minimal effort.
In contrast, polar molecules, such as water and ammonia, experience dipole-dipole interactions. These forces, akin to tiny magnets pulling on each other, create a stronger bond between molecules. As a result, polar substances exhibit higher boiling points, as their molecules require more energy to overcome the attractive forces holding them together.
The strength of intermolecular forces also impacts the boiling point of isomers. Isomers are molecules that have the same molecular formula but differ in their structural arrangement. For example, butane and isobutane both have the formula C4H10, but they have different boiling points due to differences in their intermolecular forces. Butane, with its linear structure, exhibits weaker intermolecular forces than isobutane, which has a more compact, branched structure. Consequently, butane has a lower boiling point than isobutane.
The influence of intermolecular forces on boiling point is a testament to the intricate interplay between the microscopic constituents of matter. By understanding the nature of these forces, we gain insights into the behavior of liquids and their transition into the gaseous phase.
Role of Molecular Weight in Determining Boiling Point
In the realm of chemistry, understanding the boiling point of substances is crucial. It’s the temperature at which a liquid transforms into a vapor at a given pressure, typically the standard pressure of 1 atmosphere. One key factor that significantly influences boiling point is molecular weight.
The Relationship
Molecular weight, measured in atomic mass units (amu), represents the sum of the masses of all atoms in a molecule. Generally, higher molecular weight compounds possess higher boiling points compared to their lighter counterparts. This observation stems from the stronger intermolecular forces present in heavier molecules.
Intermolecular Forces and Molecular Weight
Intermolecular forces, such as van der Waals forces, hydrogen bonding, and dipole-dipole interactions, hold molecules together in the liquid state. These forces require energy to overcome, and molecules with stronger intermolecular forces require more energy to break free and vaporize. Since molecular weight directly correlates with the number of atoms in a molecule, heavier molecules generally have more electrons, leading to stronger intermolecular forces.
Examples and Applications
Consider two alkanes: propane (C3H8) and hexane (C6H14). Propane, with a molecular weight of 44 amu, has weaker intermolecular forces and a lower boiling point (-42°C) compared to hexane, with a molecular weight of 86 amu and stronger intermolecular forces. This difference in boiling points is attributed to the increased molecular weight of hexane, which results in more van der Waals forces between its molecules.
Understanding the relationship between molecular weight and boiling point is essential in various fields. For instance, in petrochemical industries, the fractional distillation process relies on the different boiling points of hydrocarbons to separate them based on their molecular weights. Similarly, in cooking, larger molecules, such as starches, have higher boiling points and require more energy to cook compared to smaller molecules like water.
Summary
In conclusion, molecular weight plays a significant role in determining the boiling point of a compound. Heavier molecules with stronger intermolecular forces have higher boiling points, while lighter molecules with weaker intermolecular forces have lower boiling points. Understanding this relationship is vital for various applications in chemistry, industry, and everyday life.
Impact of Molecular Structure on Boiling Point
The molecular structure of a substance plays a crucial role in determining its boiling point. Symmetrical molecules with evenly distributed electrons tend to have lower boiling points than asymmetrical molecules. This is because symmetrical molecules experience weaker intermolecular forces, making it easier for them to break away from the liquid phase and enter the gas phase.
Polar molecules, in which electrons are unequally distributed, exhibit strong dipole-dipole interactions. These interactions result in stronger intermolecular forces, leading to higher boiling points. The polarity of a molecule is influenced by its electronegativity and molecular geometry. Highly electronegative atoms create a stronger dipole moment, resulting in increased polarity and higher boiling points.
Nonpolar molecules, on the other hand, have weaker van der Waals forces, which include London dispersion forces and dipole-induced dipole forces. These forces depend on the size and shape of the molecule. Larger molecules with more electrons have stronger London dispersion forces, while molecules with permanent dipoles have stronger dipole-induced dipole forces. Consequently, larger and more polar nonpolar molecules tend to have higher boiling points.
For instance, hexane, a nonpolar hydrocarbon with six carbon atoms, has a boiling point of 69 °C. In contrast, 1-hexanol, a polar alcohol with the same molecular weight, has a boiling point of 157 °C due to the presence of a hydroxyl group that introduces polarity.
In summary, the molecular structure of a substance, including its symmetry, polarity, and size, significantly affects its boiling point. Understanding these factors is essential for predicting and controlling the behavior of liquids in various applications.
The Impact of Atmospheric Pressure on Boiling Point
Imagine this: You’re preparing a delicious meal, and you’re about to boil some water for your pasta. As you place the pot on the stove, you notice that the water doesn’t start bubbling as quickly as usual. What’s going on? The culprit? The atmospheric pressure.
Atmospheric pressure is the force exerted by the weight of the air above you. It’s like an invisible blanket that presses down on everything on Earth’s surface. This pressure affects many things, including the boiling point of liquids.
The higher the atmospheric pressure, the higher the boiling point of a liquid. This is because the increased pressure makes it harder for the molecules in the liquid to escape and turn into vapor.
For example, at sea level, water boils at 100 degrees Celsius. However, if you go to a higher altitude, where the atmospheric pressure is lower, water will boil at a lower temperature. This is why cooking at high altitudes can be tricky—you need to adjust the cooking times and temperatures accordingly.
The same principle applies to other liquids as well. For instance, if you’re making coffee in the mountains, you’ll find that the coffee boils at a slightly lower temperature than it would at sea level.
Atmospheric pressure also affects the boiling point of benzene, a common solvent. At sea level, benzene boils at 80.1 degrees Celsius, but at an altitude of 1,000 meters, it boils at only 79.4 degrees Celsius.
Understanding the impact of atmospheric pressure on boiling point is crucial for various applications, including cooking, chemistry, and engineering. By adjusting the pressure, we can control the boiling point of liquids and achieve the desired results.
Vapor Pressure and Boiling Point
Unveiling the Intriguing Connection
In the realm of chemistry, boiling point holds a pivotal role, signifying the temperature at which a liquid transforms into a vapor. This phenomenon is closely entwined with the concept of vapor pressure. Join us as we explore their enigmatic connection, unlocking the secrets of equilibrium.
Vapor Pressure: A Prelude to Boiling
Imagine a liquid nestled in a closed container. Molecules within the liquid are constantly in motion, occasionally breaking free from their liquid counterparts and escaping into the gaseous phase. This process creates a layer of vapor above the liquid, exerting a force known as vapor pressure.
As temperature rises, the kinetic energy of the molecules increases, causing more frequent escapes into the vapor phase. Consequently, the vapor pressure increases. This surge in vapor pressure drives the liquid towards its boiling point.
Equilibrium: The Dance of Evaporation and Condensation
At a specific temperature, the opposing forces of evaporation (liquid to gas) and condensation (gas to liquid) reach a delicate balance, establishing equilibrium. The vapor pressure at this equilibrium point is known as the equilibrium vapor pressure.
Boiling Point: A Symphony of Equilibrium and External Pressure
The boiling point of a liquid occurs when its vapor pressure equals the external pressure exerted on the liquid. At this critical juncture, vapor bubbles can freely form within the liquid, propelling them to the surface and bursting into the vapor phase.
Interplay of Temperature and Pressure
Temperature and external pressure play a profound role in determining the boiling point. Increasing temperature elevates the kinetic energy of molecules, amplifying their escape from the liquid, and thus raising the boiling point. Conversely, decreasing external pressure lowers the boiling point, as the vapor bubbles encounter less resistance to escape.
Implications and Applications
Understanding the connection between vapor pressure and boiling point has far-reaching implications. It aids in predicting the behavior of liquids, designing distillation processes, and comprehending the boiling phenomenon. From cooking to industrial processes, the knowledge of boiling points empowers us to optimize efficiency and achieve desired outcomes.
Vapor pressure and boiling point dance in harmony, revealing the intricate nature of liquid-gas transitions. By understanding their interplay, we gain insights into the behavior of matter and unlock the potential for myriad applications, shaping our world in profound ways.
Temperature and Boiling Point
The boiling point of a substance is the temperature at which its vapor pressure equals the atmospheric pressure. This means that at the boiling point, the liquid can vaporize and turn into a gas even without being heated any further. For any given substance, the relationship between temperature and boiling point is linear.
As the temperature of a liquid increases, so does its vapor pressure. This is because the molecules of the liquid are moving faster and colliding with each other more frequently at higher temperatures. When a molecule collides with another molecule, it may gain enough energy to break away from the liquid and become a gas.
The rate at which the vapor pressure of a liquid increases with temperature depends on several factors, including:
- The strength of the *intermolecular forces between the molecules
- The molecular weight of the substance
- The molecular structure of the substance
Substances with strong intermolecular forces, such as water, have a higher boiling point than substances with weak intermolecular forces. This is because more energy is required to break the intermolecular forces and allow the molecules to escape from the liquid.
Substances with a higher molecular weight also have a higher boiling point than substances with a lower molecular weight. This is because heavier molecules have more mass and are therefore harder to move.
Substances with a more complex molecular structure also have a higher boiling point than substances with a simpler molecular structure. This is because more complex molecules have more surface area, which allows them to form more intermolecular forces.
The boiling point of a liquid also depends on the atmospheric pressure. At lower atmospheric pressure, liquids have a lower boiling point. This is because there is less pressure pushing down on the liquid, making it easier for the molecules to escape.
Vapor pressure is the pressure exerted by the vapor of a liquid when it is in equilibrium with its liquid phase. The vapor pressure of a liquid increases with temperature and is equal to the atmospheric pressure at the liquid’s boiling point.
The boiling point of a liquid is the temperature at which its vapor pressure equals the atmospheric pressure. The relationship between temperature and boiling point is linear, and the rate at which the vapor pressure of a liquid increases with temperature depends on several factors, including the strength of the intermolecular forces, the molecular weight, and the molecular structure of the substance.
Factors Affecting Benzene’s Boiling Point
Benzene is an aromatic hydrocarbon that finds widespread use as a solvent, a precursor to other chemicals, and a component of fuels. Its boiling point, the temperature at which it transforms from a liquid to a gas, is a crucial property that influences its behavior and applications. Several factors govern benzene’s boiling point:
-
*Intermolecular Forces: Benzene molecules interact through weak van der Waals forces. These forces are relatively weak compared to other types of intermolecular interactions, contributing to benzene’s low boiling point of 80.1 °C.
-
*Molecular Weight: Benzene has a molecular weight of 78.1 g/mol. Generally, as molecular weight increases, boiling point tends to increase. However, in the case of benzene, its relatively low molecular weight contributes to its lower boiling point compared to other organic compounds with similar intermolecular forces.
-
*Molecular Structure: Benzene’s unique molecular structure plays a role in its boiling point. It possesses a planar, hexagonal ring structure with alternating single and double bonds. This symmetry allows for efficient packing of molecules, reducing intermolecular forces and contributing to benzene’s lower boiling point.
Comparative Analysis of Benzene’s Boiling Point
To illustrate the influence of these factors, let’s compare benzene’s boiling point to that of similar compounds:
-
Hexane (C6H14): Hexane, an aliphatic hydrocarbon, has a higher molecular weight (86.18 g/mol) and weaker intermolecular forces than benzene. Despite its higher molecular weight, hexane’s boiling point is 13.9 °C higher than benzene, reflecting the weaker intermolecular forces in benzene.
-
Cyclohexane (C6H12): Cyclohexane, a cyclic hydrocarbon, resembles benzene in its molecular weight (84.16 g/mol) and symmetrical structure. However, cyclohexane has stronger van der Waals forces due to its larger surface area. Consequently, cyclohexane has a higher boiling point (80.7 °C) than benzene.
These comparisons highlight the interplay between molecular weight, intermolecular forces, and molecular structure in determining the boiling point of benzene.
Applications and Importance of Understanding Boiling Point
Understanding the concept of boiling point is crucial in various fields of science and engineering. It plays a significant role in numerous practical applications, from everyday household tasks to complex industrial processes.
Chemistry and Chemical Industries:
Chemists use boiling point as a key property to identify and characterize compounds. By comparing the boiling points of substances, scientists can determine their molecular structure, identify impurities, and even purify compounds through distillation. This knowledge is essential in the production of pharmaceuticals, plastics, and other chemicals.
Cooking and Food Preparation:
Boiling point has a direct impact on cooking times and techniques. In cooking, boiling is a method of heating food in a liquid, usually water. The boiling point of water at sea level is 100°C (212°F). Knowing this boiling point allows us to accurately control cooking temperatures. For example, simmering (boiling just below the boiling point) preserves the delicate flavors of certain foods, while deep-frying (boiling oil at a higher temperature) creates a crispy exterior and fluffy interior.
Engineering and Industry:
In engineering, boiling point is a critical factor in the design of heat exchangers and cooling systems. By considering the boiling points of fluids, engineers can optimize heat transfer efficiency and prevent overheating in engines, power plants, and other industrial applications.
Environmental Science:
Understanding boiling points is essential in understanding the behavior of pollutants and contaminants in the environment. For instance, the boiling point of mercury is 357°C (675°F). This information is used in designing safety protocols for handling and storing mercury to minimize the risk of exposure.
Medicine and Pharmaceuticals:
In medicine, the boiling point of solvents is important in the extraction and purification of drugs and active ingredients from plants and other sources. By knowing the boiling points of different solvents, scientists can select the most appropriate one for a given extraction process.
The boiling point is a fundamental property of matter that has a wide range of applications in various scientific and practical fields. From chemistry and cooking to engineering and medicine, understanding boiling points enables us to optimize processes, ensure safety, and advance our knowledge of the world around us.