Unveiling The Forces That Govern Biological Membrane Stability: A Comprehensive Guide
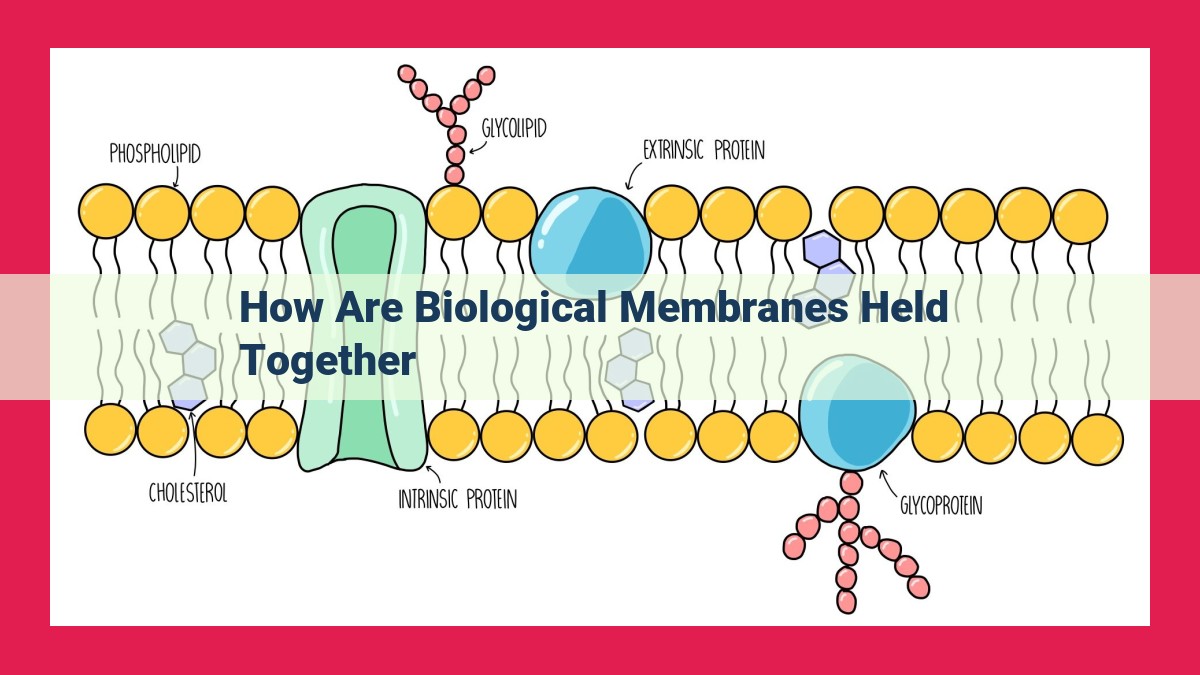
Biological membranes, essential for cellular function, are held together by a combination of forces. A phospholipid bilayer forms the membrane foundation, with hydrophilic heads facing outward and hydrophobic tails inward. Intermolecular forces such as van der Waals forces, hydrogen bonding, and covalent bonds contribute to stability. Additionally, membrane components like lipid rafts, cholesterol, and membrane proteins interact to maintain membrane fluidity, permeability, and asymmetry. Understanding membrane stability is crucial for comprehending membrane function and overall cellular processes.
Understanding the Intricate World of Biological Membranes
At the heart of every living cell lies a fundamental structure that encapsulates and protects its precious contents: the biological membrane. A delicate and complex barrier, it ensures the cell’s integrity and mediates vital processes that sustain life. Understanding the stability of this molecular fortress is paramount to unraveling the mysteries of cellular function.
The Phospholipid Bilayer: A Structural Masterpiece
Imagine a mosaic of tiny lipid molecules, arranged in a precise two-layer structure. This is the phospholipid bilayer, the backbone of biological membranes. Each lipid molecule consists of a water-loving (hydrophilic) head and a water-repelling (hydrophobic) tail. Like oil and water, the hydrophilic heads face outward, interacting with the aqueous environment, while the hydrophobic tails sequester themselves within, forming a protective barrier that shields the cell from its surroundings.
A Symphony of Molecules
Within the membrane bilayer, a host of other molecules dance in harmony, each playing a unique role in maintaining membrane stability. Lipid rafts, akin to tiny platforms, float within the bilayer, hosting specialized proteins involved in signaling and cell communication. Cholesterol, a rigid molecule, inserts itself into the bilayer, adding strength and resilience. Membrane fluidity, a measure of how easily the membrane molecules can move, is crucial for cellular processes like membrane transport and cell signaling. Membrane permeability, closely tied to fluidity, dictates which molecules can cross the membrane barrier, regulating the cell’s internal environment. Phospholipid asymmetry, a subtle imbalance in the distribution of lipid molecules across the membrane, plays a vital role in cell recognition and signaling.
The Intermolecular Dance
Binding the membrane molecules together are intricate forces. Van der Waals forces, weak attractions between adjacent lipid tails, contribute to the membrane’s overall structure. Hydrogen bonding, a stronger interaction between polar groups, further stabilizes the membrane. In some cases, covalent bonds form between lipids, creating a rigid and impenetrable barrier.
Interactions and Significance
The molecules within the biological membrane are not isolated entities but rather engage in a complex dance of interactions. Lipid rafts, cholesterol, membrane fluidity, phospholipid asymmetry, and membrane proteins all influence and depend on each other in a delicate ballet of molecular choreography. Understanding these interactions is crucial for deciphering how the membrane maintains its stability and orchestrates vital cellular processes.
Phospholipid Bilayer: The Bedrock of Biological Membranes
At the heart of every cell lies the biological membrane, a dynamic barrier that defines cellular boundaries and regulates essential processes. Understanding the stability of these membranes is crucial, as it underpins their ability to perform their vital functions.
The cornerstone of biological membranes is the phospholipid bilayer, a double layer of phospholipids. These molecules possess a hydrophilic (water-loving) head and a hydrophobic (water-hating) tail. When phospholipids come together, they spontaneously arrange themselves into a bilayer, with their hydrophobic tails facing inward and their hydrophilic heads facing outward.
This arrangement creates two distinct environments within the membrane: a hydrophobic core and two hydrophilic surfaces. The hydrophobic core effectively prevents the leakage of water-soluble molecules, while the hydrophilic surfaces enable the membrane to interact with aqueous environments.
The stability of the phospholipid bilayer stems from a combination of intermolecular forces:
- Van der Waals forces: Weak attractive forces between nonpolar molecules, which play a crucial role in holding the hydrophobic tails together.
- Hydrogen bonding: Strong attractive forces between highly electronegative atoms and hydrogen atoms, which contribute to the stability of the hydrophilic headgroups.
- Covalent bonds: Strongest intermolecular forces, which covalently link individual phospholipid molecules within the bilayer.
These forces act in concert to create a stable and dynamic membrane, which is essential for maintaining cellular integrity and regulating cellular processes.
Related Concepts: Unveiling the Nuances of Biological Membranes
In the realm of cells, biological membranes play a crucial role, acting as gatekeepers that regulate the movement of molecules in and out. To fully grasp the intricate workings of membranes, we must delve into related concepts that shape their stability and functionality.
Lipid Rafts: Specialized Membrane Microdomains
Imagine lipid rafts as tiny islands floating within the membrane. These specialized domains, enriched in cholesterol and certain lipids, create distinct microenvironments that facilitate essential cellular processes. They serve as docking stations for proteins involved in signal transduction, cell-cell communication, and other vital functions.
Cholesterol: The Membrane Stabilizer
Cholesterol, a steroid molecule, plays a pivotal role in membrane stability. Its rigid structure reinforces the membrane, preventing it from becoming too fluid. This controlled fluidity is crucial for maintaining membrane integrity and function.
Membrane Fluidity: Striking a Balance
Biological membranes are not rigid structures but rather exhibit a certain degree of fluidity. This fluidity, regulated by cholesterol, allows for membrane components to move laterally within the plane of the membrane. This dynamic nature is essential for membrane fusion, protein-protein interactions, and other biological processes.
Membrane Permeability: Controlling Molecular Movement
Cell membranes are not impermeable barriers but selectively permeable. They allow certain molecules to pass through, while others remain trapped. This selective permeability is vital for regulating the movement of nutrients, ions, and other essential molecules across the membrane.
Phospholipid Asymmetry: Maintaining Membrane Identity
Phospholipids, the building blocks of membranes, are asymmetrically distributed across the membrane’s two layers. This asymmetry is not random but rather plays a crucial role in cellular signaling, membrane trafficking, and cell recognition.
Membrane Proteins: Gatekeepers and Messengers
Embedded within the membrane are proteins that act as gatekeepers, controlling the movement of molecules across the membrane. They also serve as messengers, facilitating communication between cells. Membrane proteins are diverse in structure and function, contributing to the membrane’s overall versatility.
Intermolecular Forces: The Glue Holding Biological Membranes Together
Biological membranes, the gatekeepers of our cells, play a crucial role in maintaining cellular integrity and function. Understanding the stability of these membranes is paramount to unraveling the secrets of life’s building blocks.
Like intricate tapestries, biological membranes are woven together by a complex interplay of intermolecular forces. These forces act like the invisible threads that hold the membrane’s structure in place, ensuring its integrity and functionality.
Van der Waals forces, the weakest of the forces involved, are the subtle attractions between nonpolar molecules. In membranes, they arise from the temporary fluctuations in the electron distribution of phospholipids. Like gentle nudges, these forces contribute slightly to membrane stability.
Hydrogen bonding, a stronger force, forms between molecules with electronegative atoms and hydrogen atoms. Within membranes, hydrogen bonds occur between the polar head groups of phospholipids. These interactions, like miniature magnets, draw the molecules closely together, providing significant stability to the membrane.
Finally, covalent bonds, the strongest of the forces, form through the sharing of electrons between atoms. In membranes, covalent bonds are found in the backbone of phospholipids and in the interactions between membrane proteins. These bonds are the anchors that hold the membrane together with unwavering strength.
Together, these intermolecular forces work in concert to create a stable and functional biological membrane. This stability is essential for regulating membrane permeability, transporting nutrients, and transmitting signals within cells.
By understanding the importance of these forces, we can gain insights into the fundamental mechanisms that govern biological systems. Indeed, the stability of the biological membrane is a testament to the intricate interplay of the forces that shape the very fabric of life.
Interactions and Significance: Understanding the Membrane Mosaic
At the heart of biological membranes lies a complex interplay of diverse components, each contributing to the delicate balance of membrane stability. Lipid rafts, cholesterol, membrane fluidity, phospholipid asymmetry, and membrane proteins dance together in a symphonic harmony, orchestrating the life-sustaining functions of cells.
Lipid rafts emerge as specialized lipid-rich domains within the membrane bilayer, acting as platforms for signal transduction and protein sorting. Their raft-like properties, influenced by cholesterol and specific lipid species, create a microenvironment that fosters specific protein interactions.
Cholesterol, the unsung hero of membranes, plays a pivotal role in maintaining membrane fluidity. By impeding the close packing of phospholipids, cholesterol prevents the membrane from becoming too rigid, ensuring optimal membrane function.
Membrane fluidity refers to the ability of phospholipids to move laterally within the bilayer. This fluidity is essential for membrane remodeling, protein movement, and signal transduction. Lipid rafts contribute to local membrane fluidity variations, creating a dynamic and heterogeneous membrane landscape.
Phospholipid asymmetry arises from the unequal distribution of phospholipids between the two leaflets of the membrane bilayer. This asymmetry, maintained by flippases and floppases*, is crucial for membrane function and cell signaling. It **segregates specific lipids and proteins to their designated regions of the membrane.
Membrane proteins, the gatekeepers of cells, span the membrane bilayer and mediate a wide range of cellular processes. These proteins interact with specific lipids and other membrane components, forming functional complexes that regulate membrane permeability, transport, and signal transduction.
The interplay between these membrane components is dynamic and tightly regulated. Disruptions in this delicate balance can compromise membrane stability and have far-reaching implications for cellular function and health. Understanding the intricate relationships within the membrane mosaic is paramount for unraveling the secrets of cellular life and developing therapies to combat membrane-related diseases.
The Crucial Importance of Understanding Membrane Stability
In the intricate realm of cells, biological membranes serve as the gateways to life. These fluid boundaries, composed primarily of phospholipids, cholesterol, and proteins, play a pivotal role in maintaining cellular homeostasis and regulating the flow of molecules across the cell. Understanding their stability is paramount to grasping the very essence of life.
Membranes are not static barriers; they are dynamic structures constantly adapting to cellular demands. Their stability depends on a delicate interplay of intermolecular forces, including van der Waals forces, hydrogen bonding, and covalent bonds. These forces hold the phospholipids in a bilayer configuration, creating a hydrophobic core shielded by hydrophilic heads.
Lipid rafts, cholesterol, and membrane fluidity are key factors influencing membrane stability. Lipid rafts are specialized microdomains enriched in cholesterol and specific proteins. They provide platforms for cellular signaling and can affect membrane permeability. Cholesterol, embedded within the bilayer, modulates membrane fluidity, preventing it from becoming too rigid or fluid.
Phosphate asymmetry, or the uneven distribution of phospholipids across the membrane leaflets is another vital aspect of stability. It contributes to the membrane’s electrical potential and drives the transport of molecules. Membrane proteins, embedded in the bilayer, play a crucial role in membrane function, but their stability depends on the stability of the membrane itself.
Comprehending membrane stability is essential for unraveling the mysteries of membrane function and cellular processes. It paves the way for understanding how cells communicate, transport nutrients, and respond to their environment. By delving into the stability of these remarkable structures, we gain a profound appreciation for the delicate balance that sustains life.