Unlocking The Secrets Of Biomolecules: A Comprehensive Guide To Fats, Proteins, Nucleic Acids, And Polysaccharides
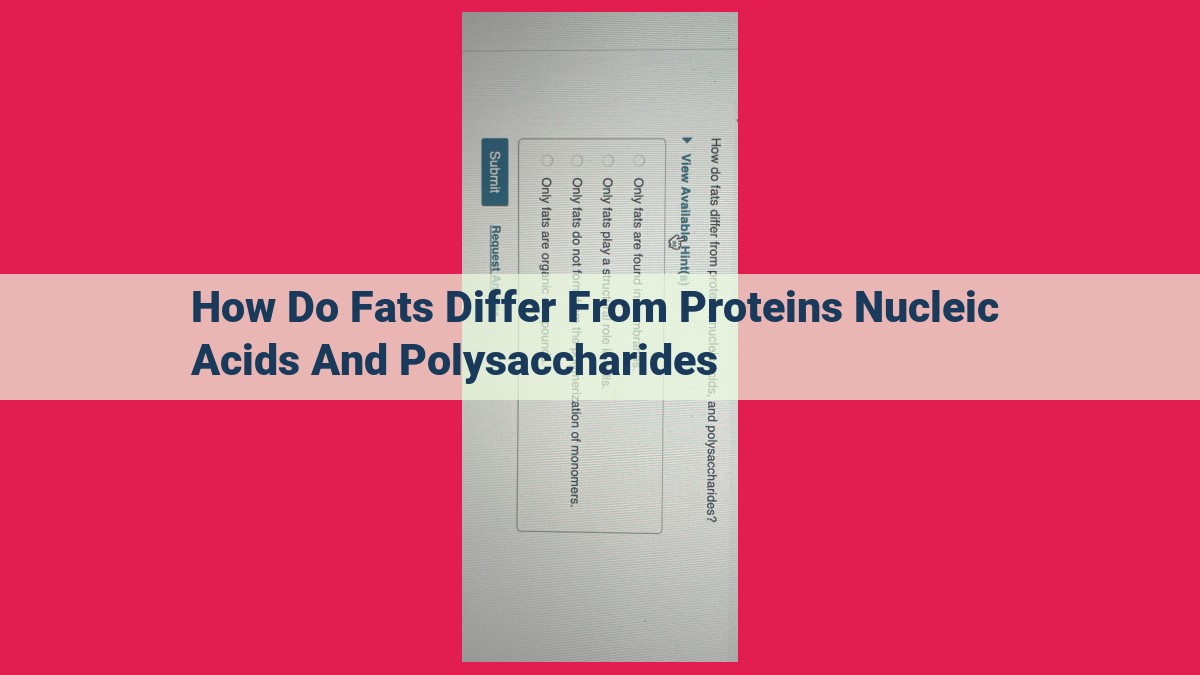
Fats, proteins, nucleic acids, and polysaccharides are major biomolecules with distinct structures. Fats are composed of fatty acids linked to glycerol, providing energy storage. Proteins are chains of amino acids, responsible for diverse cellular functions such as catalysis and structural support. Nucleic acids (DNA and RNA) carry genetic information and facilitate protein synthesis. Polysaccharides include starch and cellulose, consisting of multiple sugar units linked together for energy storage or structural roles.
A Comprehensive Guide to Matter: From Structure to Properties
Embark on a captivating journey into the realm of matter, the very essence of our physical existence. From its fundamental structure to its remarkable properties, we’ll unravel the intricate tapestry that governs the universe we inhabit.
Let’s begin our exploration by delving into the molecular structure of matter. This intricate arrangement of atoms determines the shape, size, and properties of every substance. Picture these tiny building blocks interlocking like pieces of a puzzle, defining the unique identity of each material.
Imagine a molecule of water, comprised of two hydrogen atoms and one oxygen atom. Its distinctive triangular shape and polarity allow it to form hydrogen bonds, creating the basis for life on Earth. Similarly, the carbon backbone of proteins enables them to fold into complex structures, performing essential functions within cells.
Understanding molecular structure is crucial for comprehending the behavior and interactions of matter. It governs everything from the strength of materials to the chemical reactions that drive life processes. By unraveling the intricacies of molecular structure, we unveil the secrets behind the world around us.
Arrangement of atoms, shapes, and properties of molecules
Arrangement of Atoms, Shapes, and Properties of Molecules
The molecular structure of a substance refers to the way its constituent atoms are arranged in space. This arrangement determines the shape and properties of the molecule.
Molecules can be composed of a few atoms or thousands, forming intricate structures with varying shapes. Some molecules are linear, like carbon dioxide (CO2). Others are branched, such as butane (C4H10). The _geometry_ of a molecule can affect its physical and chemical properties.
The shapes of molecules are determined by several factors, including the number and type of atoms, the presence of lone pairs of electrons, and the hybridization of the atomic orbitals. Lone pairs of electrons repel each other, influencing the arrangement of atoms and the overall shape of the molecule.
The arrangement of atoms also affects the polarity of the molecule. Polar molecules have an uneven distribution of charge, with one end being slightly positive and the other slightly negative. Nonpolar molecules, on the other hand, have an equal distribution of charge.
The shape and polarity of molecules determine their interactions with other molecules. Polar molecules tend to align with each other, forming intermolecular bonds. Nonpolar molecules, however, can only interact through weaker van der Waals forces.
Understanding the arrangement of atoms and the shape of molecules is crucial for predicting their physical and chemical properties. This knowledge has applications in various fields, including chemistry, biology, and materials science.
Composition: Unveiling the Building Blocks of Matter
Every substance in the universe, from the air we breathe to the soil we tread upon, is composed of matter. Matter can be further broken down into its fundamental building blocks: elements. These elements are the essential substances that cannot be chemically broken down into simpler substances. The vast array of matter around us is a tapestry woven from a mere 118 known elements.
Each element is characterized by a unique atomic number, which represents the number of protons in its atomic nucleus. Protons carry a positive charge, and their number determines the element’s chemical properties and its place on the periodic table. For instance, all atoms with one proton belong to the element hydrogen, while atoms with six protons form the element carbon.
In addition to protons, atoms also contain neutrons and electrons. Neutrons carry no charge and reside in the nucleus alongside protons, while electrons carry a negative charge and orbit the nucleus in energy levels. The number of neutrons and electrons in an atom can vary, giving rise to isotopes and ions of the same element.
When elements combine, they form compounds. Compounds are substances composed of two or more different elements chemically bonded together in a fixed ratio. For example, water is a compound formed from two hydrogen atoms and one oxygen atom. The properties of a compound differ significantly from those of its constituent elements, creating the vast diversity of substances we encounter in nature.
The study of the elemental composition of matter is a crucial aspect of chemistry. By understanding the elements that make up a substance, scientists can gain insights into its properties, reactivity, and potential applications. This knowledge forms the foundation for countless fields, from medicine to materials science, and underpins our understanding of the world around us.
Composition: Unraveling Matter’s Elemental Tapestry
From the smallest particles to the grandest structures, matter finds its identity in the very elements that compose it.
Atoms, the fundamental building blocks of matter, organize themselves into molecules, the complex units that make up substances. Within these molecules, elements hold sway, each contributing its unique properties and characteristics.
The proportions of these elements tell a story, revealing the substance’s composition. Imagine a recipe for a cake. Different ratios of flour, sugar, and eggs create distinct textures and flavors, shaping the final masterpiece. In a similar way, the proportions of elements determine a substance’s physical and chemical properties.
Consider iron, a metal known for its strength. Its abundance in the iron ore hematite gives the mineral its characteristic reddish-brown hue. Conversely, the presence of oxygen in iron creates iron oxide, a flaky substance used to give rust its familiar color.
The identities of elements also play a pivotal role. Sodium and chlorine, when combined, form sodium chloride, the salt we sprinkle on our food. Its distinctive taste arises from the interplay of these specific elements, creating a flavor that is both familiar and essential.
From the glittering stars above to the earth beneath our feet, matter takes on an infinite variety of forms, each with its own unique composition. Exploring these elemental proportions and identities unlocks the secrets of matter, revealing its hidden wonders and shaping our understanding of the world around us.
Molecular functions within cells
Molecular Functions Within Cells: The Busy Beehive of Life
Within the bustling microminiature world of cells lies a intricate symphony of molecules, each playing a unique and vital role. Like tiny workers in a beehive, they perform specialized tasks that together sustain the very fabric of life.
Enzymes, the maestro of cellular reactions, guide the chemical dances that convert nutrients into energy, build new structures, and dispose of waste. Hormones, the messengers of the body, transmit crucial signals between cells, organs, and tissues, coordinating their actions like a well-oiled symphony.
Transport molecules act as couriers, carrying essential molecules across cell membranes and throughout the body. They ensure that each cell receives the nutrients, oxygen, and other substances it needs to thrive. Structural proteins, like pillars of a mighty castle, provide cells with their shape and support, enabling them to maintain their integrity and carry out their functions.
Receptors, the guardians of the cell, stand poised to receive external signals. They bind to specific molecules, triggering a cascade of events that relay messages to the cell’s interior, influencing its behaviour and response to its surroundings. Storage molecules act as nature’s pantry, safeguarding precious energy (such as glucose and lipids) and genetic information (like DNA and RNA) for future use.
Antigens, the body’s vigilant sentinels, adorn the surface of cells. They serve as identifiers, allowing the immune system to distinguish between friend and foe, enabling it to mount an effective defense against infection and disease.
Physiological Functions of Cells and Organs: The Symphony of Life
Within the intricate tapestry of life, cells and organs play pivotal roles, orchestrating the symphony of our physical existence. Each cell, a microscopic marvel, houses countless biochemical reactions and processes that sustain our very being. These cells, in turn, collaborate to form organs, specialized structures that perform specific functions essential for our survival.
Organs and Their Diverse Roles
The heart, a tireless pump, propels oxygen-rich blood throughout our bodies, nourishing every cell. The lungs, delicate and sponge-like, facilitate the exchange of gases, extracting life-sustaining oxygen and expelling carbon dioxide. The liver, a metabolic powerhouse, purifies the blood, detoxifies harmful substances, and produces vital proteins.
Cellular Contributors to Organ Function
At the cellular level, a multitude of processes contribute to these organ functions. Mitochondria, the energy factories of cells, generate adenosine triphosphate (ATP), the fuel that powers our cells. The endoplasmic reticulum, a network of membranes, manufactures proteins and transports them to various parts of the cell. The Golgi apparatus, acting as a cellular distribution center, modifies and packages proteins for delivery to other cells or for secretion.
Coordinated System-Level Interactions
The physiological functions of cells and organs extend beyond their individual roles. They collaborate in concert to maintain homeostasis, the delicate balance of our internal environment. The nervous system, a complex network of nerves and neurons, transmits signals throughout the body, controlling everything from muscle movement to organ function. The endocrine system, comprising glands that secrete hormones, regulates metabolism, growth, and reproduction.
Cellular and Organ Crosstalk
Cells within organs communicate with each other through a variety of mechanisms, including gap junctions and cell surface receptors. These interactions allow for coordinated responses to external stimuli and internal signals. For example, the pancreas, which produces digestive enzymes and insulin, receives signals from the small intestine to adjust enzyme secretion and insulin release based on the intake of nutrients.
Malfunctions and Disease
When cells and organs malfunction, disease can occur. A heart attack, caused by a blockage in the coronary arteries, disrupts blood flow to the heart, potentially leading to tissue damage and loss of function. Liver cirrhosis, resulting from chronic liver damage, impairs the liver’s ability to perform its vital detoxifying and metabolic roles.
Understanding the Symphony
By delving into the physiological functions of cells and organs, we gain a deeper appreciation for the miraculous complexity of the human body. This knowledge empowers us to make informed choices that support our health and well-being. It also inspires awe and wonder at the intricate ballet of life that unfolds within us every moment.
Cellular Activities and Organ-Specific Roles
Imagine matter as the vibrant tapestry of life, weaving together the intricate symphony of biological processes. Within the microscopic realm of cells, where the smallest units of life reside, matter plays a crucial role in every cellular activity.
From the bustling energy factories mitochondria to the meticulous protein assembly lines of the ribosomes, matter provides the building blocks and the fuel that powers these microscopic machines. Proteins, the workhorses of cells, orchestrate countless reactions, transport vital nutrients, and form the structural framework that gives cells their shape and rigidity.
Moving up the scale, organs take center stage as specialized units within the body, each with its unique purpose. The liver, a master detoxifier and metabolic powerhouse, utilizes matter to filter toxins from the bloodstream and convert nutrients into energy. The heart, a tireless pump, relies on the rhythmic contraction of its muscle cells, fueled by the energy stored within matter molecules.
In the realm of the nervous system, matter takes the form of neurons, the excitable messengers that transmit electrical signals throughout the body. These intricate networks allow for rapid communication and control over every bodily function, from the simple twitch of a finger to the complex processing of emotions.
Matter, the foundational substance of the universe, plays an indispensable role in every aspect of life. From the microscopic world of cells to the macroscopic realm of organs and systems, matter provides the building blocks, energy, and information that orchestrate the harmonious symphony of biological processes. Understanding the composition and properties of matter is essential for unraveling the mysteries of life and unlocking its potential benefits for human health and well-being.
System-Level Coordination and Tasks
At the system level, matter’s functions become increasingly complex and intricate. Cells, the basic building blocks of life, orchestrate a symphony of chemical reactions, each playing a specific role within the organism. This symphony is then finely tuned by organs, which perform specialized tasks such as nutrient absorption, waste elimination, and reproduction.
The body’s circulatory and nervous systems act as messengers, connecting distant parts and coordinating their functions. Hormones, produced by glands, travel through the bloodstream, carrying signals that regulate metabolism, growth, and reproduction. Together, these systems ensure that the organism operates as a cohesive unit, responding dynamically to both internal and external cues.
Molecules and cells engage in a constant dance of communication and cooperation. Proteins, for instance, facilitate chemical reactions and transport nutrients, while DNA stores genetic information that guides development and function.
On a larger scale, the body’s immune system protects against invaders, the respiratory system exchanges gases, and the muscular system enables movement. Each of these systems relies on the seamless coordination of molecules, cells, and organs, highlighting the remarkable interdependence of matter at the system level and beyond.
Energy Storage Molecules: The Powerhouses of Life
In the intricate symphony of biological processes, energy plays a pivotal role. And at the heart of energy storage lie molecules that serve as powerhouses for living organisms. These molecules, such as fats and carbohydrates, embody the essence of energy and provide the fuel for cellular activities and organ functionality.
Fats, also known as lipids, are the primary energy reserves in our bodies. They store a massive amount of energy – twice as much as carbohydrates and proteins. This energy is released when the fats are broken down in a process called cellular respiration. Fats are also essential for cell structure and function, providing insulation, cushioning, and transporting fat-soluble vitamins.
Carbohydrates, on the other hand, are the primary source of energy for the brain and muscles. They provide glucose, the body’s preferred energy currency. When carbohydrates are digested, they are broken down into glucose, which is then utilized by cells for immediate energy production. Excess glucose can be stored in the liver and muscles as glycogen, which can be readily converted back to glucose when energy is needed.
Data storage in DNA and RNA
Data Storage in DNA and RNA: The Molecular Archives of Life
Within the enigmatic depths of living cells lies a remarkable tale of information preservation, masterfully orchestrated by the molecules of life: DNA and RNA. These nucleic acids serve as the blueprints and archives of our biological existence, encoding the blueprint of every cell and every living organism.
DNA: The Blueprint of Life
Deoxyribonucleic acid, or DNA, is the genetic material that resides in the nucleus of every cell. It’s a double helix, a twisted ladder-like structure with two strands of nucleotides winding around each other, like two entwined vines. These nucleotides, composed of a sugar backbone, a phosphate group, and one of four nitrogenous bases (adenine, thymine, cytosine, and guanine), form the letters of the genetic code.
DNA’s double-stranded nature provides redundancy, ensuring that the genetic information is not easily lost. When a cell divides, each new cell receives a complete copy of the DNA, enabling the faithful transmission of genetic information from generation to generation.
RNA: The Messenger and Interpreter
Ribonucleic acid, or RNA, is a versatile molecule that plays multiple roles in gene expression. Unlike DNA, RNA is single-stranded and more flexible. It serves as a messenger, carrying the genetic instructions from DNA out of the nucleus into the cytoplasm, where proteins are synthesized.
There are different types of RNA, each with a specific function: messenger RNA (mRNA) carries the genetic code for protein synthesis; ribosomal RNA (rRNA) forms the core of ribosomes, the cellular machinery that assembles proteins; and transfer RNA (tRNA) brings the appropriate amino acids to the ribosome for protein assembly.
Information Storage and Retrieval
The sequence of nucleotides in DNA and RNA encodes the genetic information that determines the structure and function of proteins and other molecules. The arrangement of these nucleotides is akin to a vast library of books, with each gene representing a specific chapter.
To access and interpret this genetic information, cells employ a sophisticated molecular machinery. Enzymes, like molecular translators, read the nucleotide sequence and transcribe it into RNA, which is then translated into proteins.
Implications for Life and Health
The data stored in DNA and RNA is not static but can change over time through mutations. These alterations, sometimes beneficial, sometimes detrimental, contribute to genetic diversity and enable organisms to adapt to their environment.
Understanding the intricacies of data storage in DNA and RNA is crucial for unraveling the mysteries of life, from disease diagnosis to drug development. Advances in genomics and molecular biology have revolutionized healthcare and agriculture, offering unprecedented insights into the molecular basis of life and unlocking new possibilities for improving human health and well-being.
Information Storage and Transmission in Proteins and Hormones: The Molecular Messengers
In the realm of matter, proteins and hormones play a pivotal role in communication and information management within living organisms. These remarkable molecules act as messengers, carrying crucial information that governs cellular activities, organ functions, and even systemic coordination.
Proteins, the building blocks of life, are long chains of amino acids that fold into intricate three-dimensional shapes. These intricate structures provide a unique code for each protein, enabling it to perform specific tasks. Some proteins, known as membrane receptors, reside on the surface of cells, acting as gateways that receive signals from outside the cell. Others, such as transcription factors, enter the nucleus and regulate the activity of genes, determining which proteins are produced and when.
Hormones, on the other hand, are chemical messengers that are produced in one part of the body and travel through the bloodstream to target cells in other parts of the body. They carry information about the state of the body, triggering specific responses in target cells. For example, the hormone insulin signals cells to take up glucose from the bloodstream, regulating blood sugar levels.
Proteins also play a crucial role in storing and transmitting information within cells. Enzymes, for instance, facilitate chemical reactions that are essential for cell function. Their active sites are precisely shaped to bind to specific molecules and catalyze reactions, speeding them up. Other proteins, such as immunoglobulins, are responsible for recognizing and binding to specific antigens, triggering an immune response.
Furthermore, proteins and hormones are involved in transmitting information across generations. DNA, the molecule of life, is a chain of nucleotides that encodes genetic information. Proteins called histones bind to DNA, condensing it into a compact structure called chromatin. Chemical modifications of histones can alter the accessibility of DNA, regulating gene expression and influencing the traits inherited by future generations.
Thus, proteins and hormones are essential molecular messengers that store, process, and transmit information within and between cells, regulating vital biological functions and shaping the destiny of living organisms.
Location: Mapping Matter’s Place
Every piece of matter has a specific location, from the smallest subatomic particles to the vast cosmic structures. Understanding the precise positioning of matter is crucial for comprehending its behavior and function.
At the cellular level, molecules and organelles occupy distinct compartments within the cell. For instance, DNA resides in the nucleus, while mitochondria generate energy in the cytoplasm. This spatial organization allows for efficient cellular processes.
Moving outward, the subcellular level delves into the intricate arrangements within organelles. Ribosomes, responsible for protein synthesis, are scattered throughout the cytoplasm, while Golgi bodies modify and package molecules in preparation for export.
At the tissue level, cells assemble into specialized groups to perform specific tasks. Muscle tissue, for example, consists of elongated cells that can contract, while epithelial tissue forms protective barriers lining organs.
The organ level showcases the coordinated functioning of tissues. The liver filters toxins from the blood, the heart pumps blood throughout the body, and the brain controls the body’s systems. Each organ’s location and connectivity enable efficient communication and cooperation.
Finally, the system level encompasses the interplay of organs to fulfill bodily functions. The digestive system, for example, processes food, while the circulatory system transports nutrients and oxygen. This hierarchical organization allows for the coordinated functioning of the entire organism.
Understanding the location of matter at various scales is essential for unraveling its properties, function, and interactions within living systems. From the smallest molecules to the largest organs, the precise positioning of matter orchestrates the intricate tapestry of life.
Location: Mapping Matter’s Place
Uncovering the Fabric of the Physical World
Just as the stars and planets occupy their distinct positions in the cosmos, matter, too, exists in a meticulously orchestrated arrangement within the realms of our universe. From the _cellular level to system-wide networks, the precise location of matter dictates its function, interactions, and ultimately, the very essence of life itself.
Navigating the Cellular Landscape
Cells, the fundamental building blocks of all living organisms, are microcosms of organized matter. Within these microscopic confines, matter assumes diverse _subcellular locations, fulfilling specialized roles. The _nucleus, for instance, houses _DNA, the blueprint of genetic information. _Mitochondria serve as energy powerhouses, while _ribosomes are protein synthesis factories.
Beyond the Cell’s Borders: From Tissues to Systems
As matter transcends the cellular level, it continues to exhibit an intricate hierarchy of organization. _Tissues are groups of similar cells working together to perform specific functions. Organs, composed of multiple tissues, carry out more complex tasks, such as digestion or respiration. Finally, _systems, the largest level of organization, integrate the functions of multiple organs to maintain _homeostasis and overall bodily function.
Spatial Relationships: Key to Matter’s Function
The spatial arrangement of matter is not merely a passive feature but an active determinant of its properties and interactions. The juxtaposition of cells within tissues influences their communication and cooperative behavior. The distance between molecules affects their reactivity and the formation of bonds. _Membrane structures define the boundaries of cells and organelles, controlling the movement of substances across their barriers.
Unveiling Hidden Connections
Understanding the location of matter provides invaluable insights into processes that govern the living world. By unraveling the spatial relationships within cells, tissues, and organisms, scientists can illuminate the intricate mechanisms that sustain life and elucidate the enigmatic tapestry of the physical world.
A Comprehensive Guide to Matter: Properties and Functionality
As we delve deeper into the realm of matter, we encounter a fascinating array of properties that define its reactivity, stability, flammability, corrosiveness, and toxicity. Understanding these characteristics is crucial for comprehending the behavior and applications of different substances.
Reactivity and Stability: The Dance of Chemistry
Reactivity measures how readily a substance participates in chemical reactions. Some substances, like sodium, react explosively with water, while others, like gold, remain relatively inert. This property is fundamental in chemistry, as it governs how substances interact and transform.
In contrast, stability refers to a substance’s resistance to change. Stable substances maintain their chemical structure, while unstable substances tend to decompose or react spontaneously. Understanding stability is essential for handling and storing chemicals safely.
Flammability: A Fire’s Fuel
Flammability quantifies how easily a substance catches fire and sustains combustion. Highly flammable substances, such as gasoline, burn rapidly, releasing large amounts of heat. This property has significant implications for safety and energy production.
Corrosiveness: Eating Away at Surfaces
Corrosiveness describes a substance’s ability to damage other materials through chemical reactions. Acids, for instance, are corrosive to metals, while bases can corrode organic matter. Understanding corrosiveness is crucial for selecting appropriate materials in engineering and everyday life.
Toxicity: A Matter of Health
Toxicity measures the potential of a substance to cause harm to living organisms. Some substances, like cyanide, are highly toxic, while others may be relatively harmless. Toxicological studies are essential for assessing the safety of chemicals and protecting human health.
In conclusion, these properties of matter provide a deeper understanding of its behavior and applications. By comprehending reactivity, stability, flammability, corrosiveness, and toxicity, we gain greater control over the materials we encounter, paving the way for advancements in science, technology, and daily life.
A Comprehensive Guide to Matter: From Structure to Properties
Chemical Properties: Reactivity and Stability
The chemical properties of a substance determine how it interacts with other substances and its potential for change. Reactivity refers to the ability of a substance to participate in chemical reactions, while stability refers to its resistance to change.
Understanding the potential interactions and hazards of matter is crucial in various fields. For instance, in chemistry, it helps predict the products of reactions and prevent hazardous interactions. In medicine, it guides drug development and safe handling of pharmaceutical compounds. In environmental science, it aids in assessing the impact of chemicals on ecosystems and developing remediation strategies.
Key factors influencing reactivity include the type of atoms, molecular structure, and environmental conditions. Stable substances generally have strong bonds and are unreactive. Reactive substances, on the other hand, often have weak bonds and are prone to forming new compounds.
Examples of highly reactive substances include alkali metals like sodium and potassium, which readily react with water and oxygen to produce flammable compounds. In contrast, noble gases like helium and argon are highly stable and rarely participate in chemical reactions.
Understanding chemical properties helps ensure safe handling and storage of materials, prevent accidents, and develop new applications in various industries.
Physical Properties: Defining Matter’s Behavior
In the vast expanse of matter that surrounds us, physical properties stand as key identifiers that distinguish one substance from another. These characteristics provide valuable insights into the behavior of matter and play a crucial role in countless scientific endeavors and everyday applications.
Melting Point and Boiling Point: Transformations of State
The melting point marks the temperature at which a solid transitions into a liquid state. When heat is applied to a solid, the molecules gain energy and overcome the attractive forces holding them together, causing the substance to melt. Conversely, the boiling point signifies the temperature at which a liquid transforms into a gas. At this point, the molecules acquire sufficient energy to break free from their liquid bonds and vaporize.
Density: A Measure of Compactness
Density quantifies the mass of a substance per unit volume. This property reflects the compactness of matter, with denser substances packing more mass into a smaller volume. Density variations influence buoyancy, stratification, and fluid flow dynamics.
Viscosity: Resistance to Flow
Viscosity measures the resistance of a fluid to flow. Fluids with low viscosity, such as water, flow easily, while highly viscous fluids, like honey, resist movement. This property is essential for lubrication, damping, and the behavior of fluids in pipes and channels.
Conductivity: Facilitating the Transfer of Energy and Information
Conductivity quantifies a substance’s ability to transfer heat, electricity, or sound waves. Good conductors allow for efficient transmission, while poor conductors impede it. Electrical conductivity is crucial for energy distribution and electronic devices, thermal conductivity determines the rate of heat transfer, and sound conductivity enables us to hear and perceive music.
Understanding Matter’s Physical Properties: Quantifying and Describing Characteristics
Every object, substance, and even our very bodies are composed of matter. Understanding its physical properties is crucial in unraveling its behavior and interactions in the world around us.
Physical properties are measurable, observable characteristics of matter that do not involve any chemical changes or alterations. They provide valuable insights into the nature and behavior of substances. Let’s delve into some of the most common physical properties:
-
Melting point: The temperature at which a solid transitions to a liquid state. This property indicates the strength of intermolecular forces within the substance.
-
Boiling point: The temperature at which a liquid transforms into a gas or vapor. It reflects the ease with which molecules can escape into the gaseous phase.
-
Density: The mass of a substance per unit volume. Density helps determine the buoyancy and compressibility of matter.
-
Viscosity: The resistance of a fluid (liquid or gas) to flow. High viscosity indicates strong intermolecular forces and hinders fluid movement.
-
Conductivity: The ability of a substance to transport heat, electricity, or sound. Conductivity is essential in understanding electrical circuits, heat transfer, and sound propagation.
These physical properties provide quantitative and qualitative information about matter. They help us classify substances, predict their behavior in different conditions, and design materials with specific properties. By understanding these characteristics, we gain a deeper appreciation for the diversity and complexity of the material world around us.
Molecular mass, molar mass, relative molecular mass, and formula weight
Molecular Mass: Weighing the Giants of Matter
In the realm of chemistry, the concept of mass plays a pivotal role. Molecular mass, a fundamental property of substances, sheds light on the weight of these tiny particles. It encapsulates the combined mass of all the atoms within a molecule, allowing scientists to compare the relative heaviness of different compounds.
Molecular mass is measured in Daltons (Da), named after the renowned chemist John Dalton. Each Dalton represents the mass of one atomic mass unit (amu), which is approximately equal to the mass of a single proton. For instance, the molecular mass of water (H2O) is 18 Da, as it consists of two hydrogen atoms (each with a mass of 1 Da) and one oxygen atom (with a mass of 16 Da).
Molar Mass: Unveiling the Giant’s Masses
Molar mass, closely linked to molecular mass, takes into account the number of molecules present in a given quantity of a substance. It is expressed in grams per mole (g/mol), where a mole represents a specific number of particles (6.022 x 10^23).
By determining the molar mass, scientists can calculate the mass of a specific amount of a substance. For instance, the molar mass of sodium chloride (NaCl) is 58.44 g/mol. This tells us that one mole of sodium chloride weighs 58.44 grams.
Relative Molecular Mass: A Comparative Scale
Relative molecular mass, as its name suggests, compares the molecular mass of a substance to that of a reference substance. This reference is usually carbon-12, which has a relative molecular mass of exactly 1.
Relative molecular mass is particularly useful in determining the molecular formula of a compound. By comparing the experimental molecular mass of a compound to its relative molecular mass, chemists can deduce the number and types of atoms present in the molecule.
Formula Weight: A Shortcut for Molar Mass
Formula weight, often used interchangeably with molar mass, is a term particularly relevant to ionic compounds. These compounds consist of positively charged ions (cations) and negatively charged ions (anions) that combine to form a neutral compound.
The formula weight of an ionic compound is calculated by adding the atomic masses of the constituent atoms, accounting for their relative proportions. This provides a quick estimate of the molar mass of the compound.
By delving into the intricate realm of molecular, molar, and formula mass, scientists gain a deeper understanding of the building blocks of matter and their interactions. These concepts serve as essential tools in deciphering the complexities of the chemical world.
Molecular Weight: Measuring and Comparing the Mass of Molecules
Imagine matter as a vast universe of tiny building blocks, each with a precise mass. To navigate this microscopic realm, scientists use a fundamental concept: molecular weight. It’s the yardstick that allows us to measure and compare the mass of molecules.
Molecular mass represents the total mass of all the atoms in a single molecule, expressed in atomic mass units (amu). To determine the molecular mass of a compound, we simply add up the atomic masses of each element, multiplied by the number of atoms present in the formula.
For instance, let’s consider water (H2O). Each water molecule contains two hydrogen atoms and one oxygen atom. The atomic mass of hydrogen is approximately 1 amu, while the atomic mass of oxygen is about 16 amu. Thus, the molecular mass of water is 18 amu (2 amu for hydrogen + 16 amu for oxygen).
Comparing molecular weights is crucial in various scientific disciplines. In chemistry, it helps us predict the properties of compounds, such as their reactivity and solubility. In biology, it aids in understanding the function of biomolecules, such as proteins and DNA.
Molar mass is another important concept related to molecular weight. It represents the mass of one mole of a substance, which is approximately 6.022 x 10^23 particles. Molar mass is measured in grams per mole (g/mol) and is often used to convert between the mass and the number of particles in a sample.
By understanding molecular and molar mass, we can quantify the mass of molecules and compare their properties. This knowledge empowers scientists to explore the intricate world of matter and unravel its mysteries.
Dive into the Enchanting Realm of Matter: A Tale of Shapes
Unleashing the Secrets of Molecular Geometry
The intricate dance of atoms within molecules reveals a mesmerizing array of shapes. These molecular shapes are not mere curiosities; they hold profound implications for the substance’s properties and behaviors. Imagine a molecule resembling a tetrahedron, with four atoms arranged at its corners, or a linear molecule, with atoms lined up like pearls on a string.
Exploring Atomic and Crystal Symmetries
Beyond molecules, atoms themselves possess distinct geometrical arrangements. Arrange these tiny building blocks in an orderly fashion, and you create crystals. Whether they form a cubic lattice, a hexagonal honeycomb, or something more exotic, crystal shapes provide clues to the internal structure and properties of the substance.
Unraveling the Intricacies of Organ Morphology
The world of shapes extends far beyond the molecular and atomic realms. Organs, the building blocks of life, display a remarkable diversity of forms. The rhythmic contractions of a heart, the intricate folds of a brain, and the delicate branching of lungs are testament to the intricate dance of matter at the organ level.
Implications and Applications
The understanding of matter’s shapes, from molecules to organs, has far-reaching implications. It enhances our ability to design new materials, develop effective drugs, and unravel the mysteries of biological processes. By grasping the geometry of matter, we unlock the secrets of its interactions, functions, and potential applications.
From the microscopic realm of atoms to the macroscopic domain of organs, the shapes of matter enchant and inform our understanding of the world around us. Through a deeper appreciation of these geometrical wonders, we not only gain knowledge but also unlock the possibilities for scientific advancements and technological breakthroughs. Embrace the captivating story of matter’s shapes, and discover the limitless possibilities that lie within its embrace.
Shape: Unveiling Matter’s Geometry
In the realm of matter, shape is a captivating attribute that orchestrates its existence. Beyond the simplistic notions of circles and squares, molecules, atoms, crystals, and even our very organs possess intricate three-dimensional arrangements that profoundly impact their properties and functions.
Imagine a symphony of molecules, each with its own unique dance. Their shapes determine how they interact, forming bonds, shaping cellular structures, and governing the synthesis of essential biomolecules. From the graceful double helix of DNA to the globular proteins that orchestrate cellular machinery, the three-dimensional architecture of matter is a masterpiece of design.
Beyond molecules, the shape of crystals mesmerizes with its kaleidoscopic patterns. Their orderly arrangements of atoms and ions create shimmering surfaces and fascinating optical properties. Diamonds owe their brilliance to their tightly packed carbon atoms, while quartz’s characteristic hexagonal prisms reflect light in captivating ways.
In the human body, the shape of an organ is more than just a matter of aesthetics. The heart’s muscular and conical structure enables it to propel blood throughout the body, while the lungs’ porous and branching architecture allows for efficient gas exchange. Every organ’s unique shape is intricately linked to its specific function.
Unraveling the three-dimensional arrangements of matter unveils an extraordinary symphony of forms, each with its own intricate purpose and implication. It is through this exploration that we gain a profound appreciation for the complexity and beauty of our material world.
Solubility: Unveiling the Mix-and-Match World of Matter
In the realm of chemistry, solubility reigns as a fascinating dance between substances and their liquid partners. This property dictates the ability of a substance to dissolve or mix evenly within a given liquid, revealing crucial insights into the molecular interactions that shape our world.
Aqueous Solubility: The Watery Dance
When it comes to aqueous solubility, water takes center stage as the solvent of life. Substances that readily dissolve in water are deemed hydrophilic, literally meaning “water-loving.” These molecules typically possess polar characteristics, forming cooperative bonds with water’s own polar molecules.
Organic Solvent Solubility: Beyond the Aqueous Realm
Organic solvents, such as ethanol and chloroform, offer a diverse range of properties for dissolving substances. Molecules with nonpolar characteristics, lacking significant charge separation, tend to dissolve favorably in nonpolar solvents like hexanes. Conversely, polar molecules prefer polar organic solvents like dimethylformamide.
Lipid Solubility: Fat-Soluble Secrets
Lipids are an essential class of molecules that form the building blocks of cell membranes and other biological structures. They are characterized by their nonpolar nature, and thus, they dissolve more readily in nonpolar solvents. This phenomenon is crucial for many biological processes, such as the transport of fat-soluble vitamins and hormones.
The Power of Solubility: Shaping Our World
Solubility plays a profound role in various aspects of our lives. From the mixing of paints and solvents to the extraction of drugs from plants, it governs the interactions between substances and the environment. Understanding solubility allows scientists, engineers, and artists to manipulate and exploit the properties of matter, creating innovative materials and advancing our knowledge of the world around us.
Tips for Maximizing Solubility
To enhance the solubility of a substance, consider the following strategies:
- Increase the temperature: Raising the temperature generally increases solubility, as the increased molecular motion favors the formation of more intermolecular interactions.
- Stir or agitate: Mechanical agitation helps disperse the substance and promote contact with the solvent, facilitating dissolution.
- Use a suitable solvent: Choosing the right solvent for the substance is crucial. Polar substances dissolve better in polar solvents, while nonpolar substances prefer nonpolar solvents.
Unveiling the Secrets of Solubility: Exploring Matter’s Interactions
In the vast realm of matter, the ability of substances to dissolve in different liquids holds profound implications for countless natural and industrial processes. Solubility, the measure of this ability, reveals the intricate dance between molecules and their surrounding environment.
Imagine a world where water, the universal solvent, reigns supreme. Its ability to dissolve a vast array of substances has shaped our planet’s landscapes, oceans, and even the very cells that sustain life. However, not all liquids are created equal. Organic solvents, such as alcohol and acetone, possess unique properties that allow them to dissolve nonpolar molecules that water cannot.
Solubility plays a pivotal role in a myriad of everyday applications. From the medications we take to the cleaning products we use, understanding the solubility of substances is crucial for safety and effectiveness. In the pharmaceutical industry, scientists meticulously design drugs with specific solubilities to ensure their delivery to targeted tissues. Similarly, in manufacturing, selecting the right solvent for a given process can optimize efficiency and product quality.
Unveiling the secrets of solubility requires delving into the molecular realm. When a substance dissolves in a liquid, its molecules interact with the solvent molecules. In the case of water, it forms hydrogen bonds with polar molecules, attracting them towards each other. Nonpolar molecules, on the other hand, interact more favorably with organic solvents, which have less polar character.
Factors such as temperature and pressure can also influence solubility. Increasing temperature generally increases solubility, while increasing pressure can either increase or decrease it depending on the substance and solvent.
Understanding solubility is key to unlocking a treasure trove of scientific and practical applications. From designing efficient cleaning products to developing life-saving drugs, the study of this phenomenon continues to pave the way for advancements in industry, medicine, and beyond.