Beer-Lambert Law: Exploring The Inverse Relationship Between Absorbance And Concentration
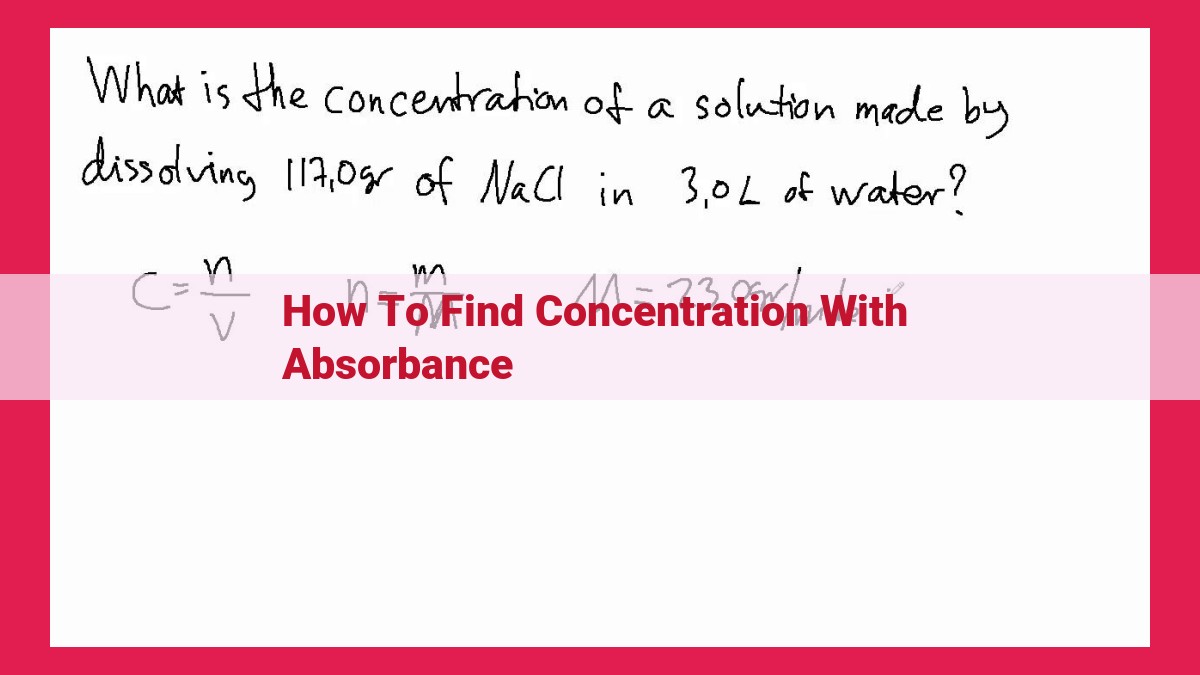
Absorbance is inversely proportional to the concentration of a solution, as described by the Beer-Lambert Law. The law establishes a linear relationship between absorbance, molar absorptivity (a constant unique to each substance), concentration, and path length. By measuring absorbance and knowing the molar absorptivity and path length, the concentration of a solution can be calculated. This principle is widely used in analytical chemistry, biochemistry, and environmental science to determine the concentration of various substances in solutions, aiding in research, quality control, and environmental monitoring.
Absorbance: An Essential Concept in Measuring Concentration
In the realm of analytical chemistry, absorbance plays a crucial role in unraveling the mysteries of solutions and their contents. Absorbance, simply put, is a measure of how much light a substance absorbs when exposed to a specific wavelength. Like a fingerprint, absorbance provides valuable insights into a substance’s identity and its concentration within a solution.
Understanding absorbance is like embarking on a scientific adventure. It’s a tool that unlocks the secrets of chemistry and has far-reaching applications in fields as diverse as medicine, environmental monitoring, and food analysis. So, let’s dive into the fascinating world of absorbance and discover its power in unveiling the mysteries of concentration.
Factors Affecting Absorbance: Delving into the Beer-Lambert Law
In the realm of chemistry and beyond, absorbance plays a pivotal role in unraveling the mysteries of solutions and their components. One of the cornerstones of understanding absorbance is the renowned Beer-Lambert Law, which provides a quantitative framework for deciphering the factors that govern this phenomenon.
The Beer-Lambert Law, elegantly expressed by the equation A = εbc, establishes a direct relationship between absorbance (A), a measure of the extent to which light is absorbed by a sample, and three other crucial parameters:
-
Molar absorptivity (ε): This is a substance-specific constant that reflects the inherent ability of a compound to absorb light at a particular wavelength. It depends on the arrangement of atoms within the molecule, providing insights into its chemical structure and properties.
-
Concentration (c): This represents the amount of solute dissolved in a solvent, usually expressed in units of moles per liter. The higher the concentration, the more molecules are present to absorb light, resulting in a higher absorbance.
-
Path length (b): This refers to the distance that light travels through the solution. It is typically measured in centimeters. As light traverses a longer path through the solution, it encounters more absorbing molecules, leading to a proportional increase in absorbance.
Understanding these factors and their interconnectedness is essential for interpreting absorbance measurements accurately. The Beer-Lambert Law allows scientists to determine unknown concentrations by measuring absorbance and utilizing the known values of molar absorptivity and path length.
So, the next time you encounter absorbance in a scientific context, remember the Beer-Lambert Law as your trusted guide. By unraveling the factors that affect absorbance, you can harness its power to unlock valuable information about the composition and properties of the world around you.
Understanding Molar Absorptivity: A Substance’s Unique Fingerprint
When light interacts with a substance, it can either be absorbed or transmitted. Absorbance is a measure of how much light is absorbed by a substance, and it can provide valuable insights into the substance’s chemical structure and properties. This is where molar absorptivity comes into play, a substance-specific characteristic that serves as its unique fingerprint.
Definition and Significance of Molar Absorptivity
Molar absorptivity (ε) is a constant that represents the ability of a substance to absorb light at a specific wavelength. It is defined as the absorbance of a solution containing 1 mole of the substance per liter of solvent, when the path length is 1 centimeter. Molar absorptivity is an intrinsic property of a substance, meaning that it is unaffected by the concentration or path length of the solution.
Relationship to Chemical Structure and Properties
The magnitude of molar absorptivity depends on the substance’s electronic structure. Specifically, it is influenced by the presence of chromophores, which are chemical groups that absorb light. The number, type, and arrangement of chromophores within a molecule determine its absorption spectrum, a graphical representation of absorbance at different wavelengths.
For example, conjugated double bonds enhance molar absorptivity, as they allow electrons to move more easily within the molecule, facilitating light absorption. Additionally, the presence of heavy atoms, such as bromine or iodine, can also increase molar absorptivity.
By analyzing the molar absorptivity and absorption spectrum of a substance, researchers can deduce information about its functional groups and molecular structure. This makes molar absorptivity a powerful tool for chemical identification, structural analysis, and quantitative analysis.
Path Length: The Distance Light Travels
The path length refers to the distance that light travels through a solution. This path length plays a crucial role in determining the absorbance of a solution.
As light passes through a solution, it interacts with the molecules present in the solution. The more molecules the light encounters, the greater the absorbance. Imagine a maze where light represents a person trying to find their way. The longer the maze path, the more likely they are to encounter obstacles and take a longer time to reach their destination. Similarly, the longer the path length, the higher the absorbance as light encounters more molecules along its journey through the solution.
Therefore, it is essential to maintain a consistent path length when measuring absorbance to ensure accurate and reproducible results. Using a cuvette with a fixed path length ensures that the amount of light absorbed is solely due to the concentration of the solution and not due to variations in the path length.
Calculating Concentration Using the Beer-Lambert Law Equation
The Beer-Lambert Law equation is a fundamental tool in analytical chemistry, providing a direct link between absorbance and the concentration of a solution. This equation enables scientists to determine the unknown concentration of a substance based on its absorbance measurements.
The Equation
The Beer-Lambert Law equation takes the form:
**Absorbance (A) = ε * l * C**
where:
- Absorbance (A) is the measured absorbance value.
- ε (molar absorptivity) is a constant that represents the light-absorbing properties of the substance at a specific wavelength.
- l (path length) is the distance the light travels through the sample.
- C (concentration) is the unknown concentration of the substance.
Units
The units of the equation are:
- Absorbance (A) is unitless.
- Molar absorptivity (ε) is expressed in L/(mol*cm).
- Path length (l) is expressed in cm.
- Concentration (C) is expressed in mol/L.
Using the Equation
To determine the concentration of a solution using the Beer-Lambert Law equation, follow these steps:
- Measure the absorbance (A) of the sample using a spectrophotometer.
- Determine the molar absorptivity (ε) of the substance at the wavelength of measurement.
- Measure the path length (l) of the spectrophotometer cell.
- Rearrange the equation to solve for concentration (C): C = A / (ε * l)
- Substitute the measured and known values into the equation and calculate the unknown concentration.
Applications
The Beer-Lambert Law equation finds widespread application in various fields, including:
- Analytical chemistry: Determining the concentration of substances in complex mixtures.
- Biochemistry: Measuring the concentration of biomolecules, such as proteins and DNA.
- Environmental science: Monitoring pollutant concentrations in water, air, and soil.
Importance
The Beer-Lambert Law equation is a powerful tool that provides a reliable and accurate method for determining the concentration of solutions. Understanding the factors that affect absorbance and correctly applying the equation ensures accurate and reproducible results.
Applications of Absorbance Measurements: Unlocking the Secrets of Concentration
Absorbance, a fundamental property of matter, plays a pivotal role in scientific investigations across diverse disciplines. Its applications span from analytical chemistry, where it aids in determining the concentration of various substances, to biochemistry, environmental science, and beyond.
Analytical Chemistry: Quantifying the Unseen
In analytical chemistry, absorbance measurements serve as an essential tool for quantifying the concentration of specific analytes in a sample. By measuring the absorbance of light at a specific wavelength characteristic of the analyte, scientists can determine its concentration using the Beer-Lambert Law equation. This technique finds applications in various fields, including medical diagnostics, pharmaceutical analysis, and food safety testing.
Biochemistry: Exploring the Molecular World
Absorbance measurements have revolutionized the field of biochemistry, providing insights into the structure and function of biological molecules. By measuring the absorbance of proteins and nucleic acids at specific wavelengths, scientists can determine their concentration and study their interactions with other molecules. This knowledge is crucial for understanding cellular processes, diagnosing diseases, and developing new therapies.
Environmental Science: Monitoring Environmental Health
In environmental science, absorbance measurements are used to monitor the concentration of pollutants in various environmental samples, such as water, air, and soil. By measuring the absorbance of light at wavelengths specific to different pollutants, scientists can assess their presence and potential impact on ecosystems and human health. This information is vital for developing environmental regulations and safeguarding the quality of our surroundings.