Bacterial Translation: Key Differences And Antibiotic Susceptibility
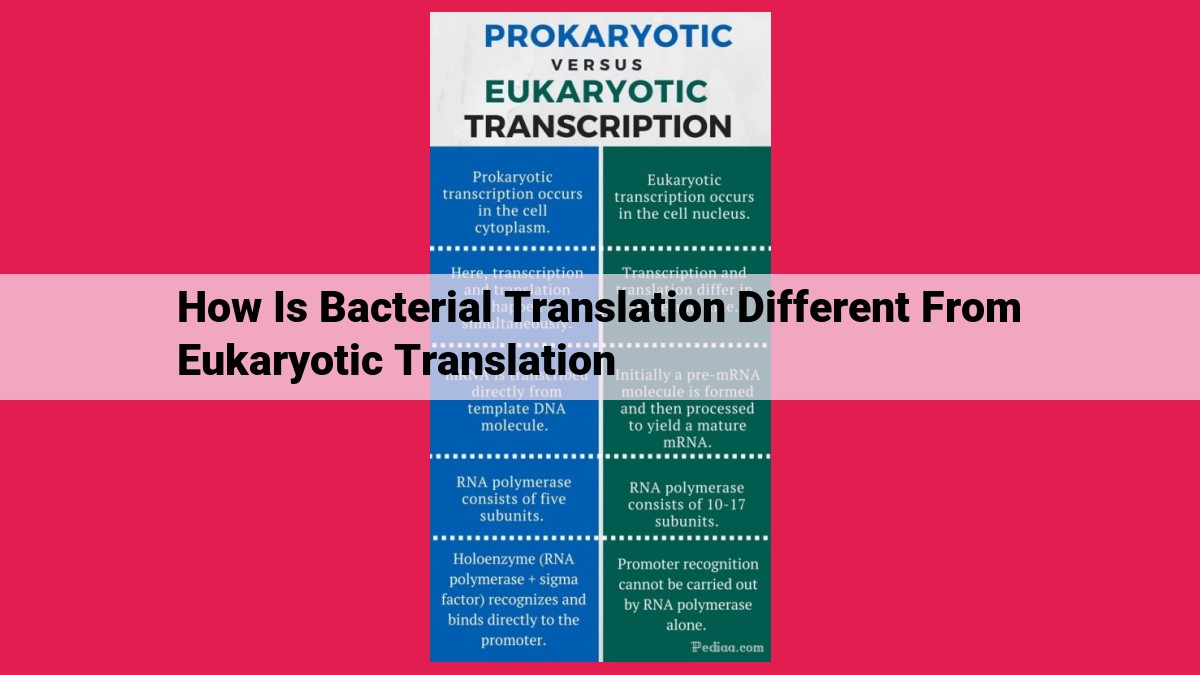
Bacterial translation diverges from its eukaryotic counterpart in several ways. Initiation involves distinct initiation factors and a smaller ribosome structure in bacteria. Bacterial tRNAs exhibit differences in sequence and structure, allowing for less stringent codon-anticodon pairing rules. Elongation and termination factors also vary, contributing to differences in protein synthesis rates and termination mechanisms. These variations make bacterial translation unique and susceptible to specific antibiotics that target initiation, elongation, or termination steps, exploiting the differences between prokaryotic and eukaryotic translation pathways.
Prokaryotic vs. Eukaryotic Translation: Unraveling the Differences
The Dance of Life: A Tale of Genetic Interpretation
In the symphony of life, translation stands as a crucial conductor, orchestrating the conversion of genetic blueprints into the proteins that orchestrate every cellular function. But beneath this shared purpose, prokaryotic and eukaryotic cells, the two fundamental realms of life, play out this dance with exquisite variations.
Prokaryotes, the simpler cousins of eukaryotes, lack the elaborate internal structures of their eukaryotic counterparts. This simplicity extends to their ribosomes, the molecular machines that decode genetic messages. Prokaryotic ribosomes are smaller and more compact, featuring two subunits, 50S and 30S, compared to the larger eukaryotic ribosomes with 60S and 40S subunits. These structural differences have profound implications for how prokaryotes and eukaryotes read the genetic code.
Moreover, the tRNAs, the adapters that carry amino acids to the ribosome, exhibit distinct characteristics between the two realms. Prokaryotic tRNAs are typically smaller and lack certain modifications found in eukaryotic tRNAs. These differences affect their recognition by ribosomes and influence the fidelity of codon-anticodon pairing, the crucial step where the genetic code is decoded.
Finally, the cast of translation factors, the proteins that orchestrate the intricate steps of translation initiation, elongation, and termination, differ between prokaryotes and eukaryotes. These factors play a crucial role in ensuring the accuracy and efficiency of protein synthesis.
Unveiling the Impact: From Antibiotics to Disease
These subtle yet profound differences between prokaryotic and eukaryotic translation have far-reaching consequences. Antibiotics, the wonder drugs that combat bacterial infections, often target specific steps in translation. Understanding these differences is essential for designing antibiotics that selectively target prokaryotic ribosomes without disrupting eukaryotic cells.
Similarly, diseases caused by genetic defects in certain translation factors can manifest differently in prokaryotes and eukaryotes. Mutations in eukaryotic translation factors, for instance, often lead to developmental abnormalities, while mutations in prokaryotic factors primarily affect bacterial growth.
Appreciation for the Distinctions
By unraveling the intricacies of prokaryotic and eukaryotic translation, scientists gain invaluable insights into the fundamental processes of life. This knowledge empowers us to understand the mechanisms of antibiotic action, develop targeted therapies for diseases, and appreciate the exquisite diversity of cellular life on Earth.
Unlocking the Secrets of Translation: Prokaryotic vs. Eukaryotic Differences
Prepare for a Journey into the Molecular Realm
As we delve into the fascinating world of translation, the process by which genetic information is converted into proteins, we embark on a journey to understand the intricate differences between prokaryotic and eukaryotic cells. These distinctions shape how they interpret the genetic code, impacting protein synthesis and cellular function.
Key Distinctions: A Tale of Two Cells
Ribosome Structure: The heart of translation, ribosomes, differ between prokaryotic and eukaryotic cells. Prokaryotic ribosomes are smaller (70S) than their eukaryotic counterparts (80S), influencing tRNA binding and codon recognition.
Initiation Factors: Initiation factors play a crucial role in starting translation. Eukaryotic cells employ a more complex set of initiation factors than prokaryotes, reflecting the increased complexity of their translation machinery.
Termination Factors: When translation reaches its end, termination factors step in. Prokaryotic and eukaryotic termination factors differ in their mechanisms, underlining the distinct termination processes in each cell type.
tRNA Structure: Transfer RNAs (tRNAs) carry amino acids to the ribosome for incorporation into proteins. While sharing general features, prokaryotic tRNAs lack the intron-containing sequences found in eukaryotic tRNAs.
Elongation Factors: Elongation factors guide the ribosomal movement along the mRNA during protein synthesis. Prokaryotic elongation factors have different structures and functions compared to their eukaryotic counterparts.
Implications for Protein Synthesis and Beyond
These fundamental differences between prokaryotic and eukaryotic translation have profound implications for protein synthesis and cellular function. They contribute to the unique biology of each cell type, influencing protein diversity, antibiotic susceptibility, and overall cellular physiology.
Understanding these distinctions is critical for unraveling the mysteries of molecular biology, advancing medical treatments, and unlocking the secrets of living systems.
The Role of Initiation Factors in Translation Initiation
Translation, the process of converting genetic information into proteins, is a fundamental cellular function. It involves the decoding of messenger RNA (mRNA) by transfer RNA (tRNA) to synthesize a polypeptide chain. Initiation, the first step of translation, is orchestrated by a group of proteins known as initiation factors (IFs).
Functions of Initiation Factors
IFs play a crucial role in recruiting the small ribosomal subunit to the mRNA and positioning the start codon (AUG). They also assist in binding the initiator tRNA (bearing methionine) to the start codon. This complex process ensures the accurate initiation of protein synthesis.
Prokaryotic and Eukaryotic IFs
Prokaryotic and eukaryotic initiation pathways differ in their complexity. Prokaryotes utilize a single IF, which has all the necessary functions for initiation. Eukaryotes, on the other hand, employ multiple IFs, each with specific roles in recruiting the ribosomal subunits and initiating tRNA binding.
Relationship to Ribosome Structure and tRNA Binding
The structure of the ribosome influences IF binding and tRNA recognition. IFs interact with specific sites on the ribosome to facilitate the correct orientation of the mRNA and tRNA. They also interact with the anticodon loop of the initiator tRNA, ensuring its proper positioning on the start codon.
Codon-Anticodon Pairing
IFs contribute to the accuracy of codon-anticodon pairing by stabilizing the interaction between the start codon and the initiator tRNA. Incorrect pairing can lead to errors in protein synthesis.
IFs are essential for initiating translation, a critical process for gene expression and protein synthesis. Understanding their functions, particularly in the context of prokaryotic and eukaryotic differences, provides insights into the fundamental mechanisms of cellular machinery.
Journey Through the Translation Process: Unveiling the Differences Between Prokaryotic and Eukaryotic Initiation Factors
In the vibrant tapestry of life, translation plays a pivotal role in translating the genetic code of mRNA into the functional proteins that orchestrate cellular processes. At the heart of this intricate process lies the ribosome, a molecular machine that orchestrates the precise assembly of amino acids. However, the journey from mRNA to protein differs between prokaryotes and eukaryotes, two fundamental domains of life, due to distinct initiation factors that govern the start of protein synthesis.
Initiation Factors: The Gatekeepers of Translation
Initiation factors are the unsung heroes of translation, ensuring the precise start of protein synthesis. They facilitate the formation of the initiation complex, a molecular handshake between mRNA, the ribosome, and the first tRNA molecule carrying the initiator amino acid. Prokaryotes, such as bacteria, utilize a set of three initiation factors (IF1, IF2, and IF3), while eukaryotes employ a more complex repertoire of 12 initiation factors.
Unveiling the Variations
IF1: This initiation factor binds to the small ribosomal subunit, preventing the premature association with the large subunit. Together with IF3, IF1 stabilizes the small subunit, creating a receptive environment for mRNA binding.
IF2: The star of prokaryotic initiation, IF2 binds to both mRNA and the initiator tRNA, guided by the Shine-Dalgarno sequence, a specific sequence present in bacterial mRNA. This interaction ensures the correct alignment of the ribosome on the mRNA, initiating the decoding process.
IF3: This initiation factor, unique to prokaryotes, stabilizes the interactions between the small and large ribosomal subunits, preventing premature dissociation during initiation.
In contrast, eukaryotic initiation factors display a greater diversity and complexity. eIF3 is a large complex that stabilizes the small ribosomal subunit and binds specific regions of the mRNA, ensuring precise initiation. eIF4A unwinds the RNA secondary structure, facilitating ribosome binding. eIF4B recruits the small ribosomal subunit, while eIF4E binds the 5′ cap of the mRNA, guiding the ribosome to the start codon.
The Significance of Differences
The variations in initiation factors between prokaryotes and eukaryotes stem from fundamental differences in their cellular structure and genetic organization. Prokaryotes lack a nucleus and possess smaller genomes, enabling a simpler initiation process. Eukaryotes, with their intricate nuclear compartments and larger genomes, require a more sophisticated initiation machinery to navigate the complexities of mRNA regulation.
The distinctions in bacterial and eukaryotic initiation factors highlight the remarkable diversity and complexity of translation. These factors play a crucial role in ensuring the precise initiation of protein synthesis, a process essential for life’s fundamental processes. Understanding these differences not only expands our knowledge of molecular biology but also provides valuable insights into the development of antibacterial drugs that target translation initiation.
Ribosome Structure and Codon-Anticodon Pairing
In both prokaryotes and eukaryotes, the ribosome serves as the protein synthesis machinery. Ribosomes are complex macromolecules composed of ribosomal RNA (rRNA) and ribosomal proteins.
The ribosome structure is crucial for facilitating codon-anticodon pairing, which is the key step in deciphering the genetic code. Codon-anticodon pairing occurs between the codons on the messenger RNA (mRNA) and the anticodon loops of transfer RNA (tRNA) molecules.
In prokaryotes, ribosomes are smaller and have a simpler structure compared to eukaryotes. They consist of two subunits, the 30S small subunit and 50S large subunit. The 30S subunit is responsible for binding the mRNA and the initiator tRNA, while the 50S subunit catalyzes the peptide bond formation.
Eukaryotic ribosomes, on the other hand, are larger and more complex. They consist of two subunits, the 40S small subunit and 60S large subunit. The 40S subunit is analogous to the 30S subunit in prokaryotes, while the 60S subunit is significantly larger and contains additional components involved in protein synthesis.
Codon-anticodon pairing is the process by which the ribosome matches the codons on the mRNA with the appropriate anticodons on the tRNA molecules. This pairing ensures that the correct amino acids are added to the growing polypeptide chain.
The specific structure of the ribosome RNA and proteins is essential for codon-anticodon pairing. The rRNA components provide the framework for the ribosome structure and contain nucleotides that interact with the codons and anticodons. Ribosomal proteins, in turn, stabilize the ribosome structure, facilitate tRNA binding, and assist in the elongation and termination steps of translation.
In summary, the ribosome structure, tRNA structure, and codon-anticodon pairing are intimately intertwined processes that ensure the accurate translation of the genetic code into proteins. Understanding these relationships is critical for comprehending the molecular basis of protein synthesis and its implications for antibiotic susceptibility.
Comparison of prokaryotic and eukaryotic ribosome structure
Comparison of Prokaryotic and Eukaryotic Ribosomes
In the intricate world of protein synthesis, ribosomes take center stage as the molecular machines responsible for translating the genetic code into the proteins that power our cells. While prokaryotic and eukaryotic cells share the fundamental principles of translation, their ribosomes exhibit distinct features that reflect their different evolutionary paths.
Size and Complexity
- Prokaryotic ribosomes: Small in size (70S ribosomes) and less complex in structure.
- Eukaryotic ribosomes: Larger (80S ribosomes) and more elaborate, consisting of two subunits, the large (60S) and small (40S) subunits.
Subunit Composition
- Prokaryotic ribosomes: Contain fewer proteins and fewer RNA molecules.
- Eukaryotic ribosomes: Composed of a greater number of proteins and rRNA molecules, resulting in a more intricate structure.
Location
- Prokaryotic ribosomes: Freely suspended in the cytoplasm.
- Eukaryotic ribosomes: Bound to the endoplasmic reticulum (ER) or free in the cytoplasm. This compartmentalization plays a role in protein targeting and secretion.
Function in Translation
- Both ribosomes: Bind mRNA and tRNA molecules, guiding the precise pairing of codons with anticodons.
- Prokaryotic ribosomes: Have a less specific codon-anticodon recognition mechanism, which can lead to wobble base pairing.
- Eukaryotic ribosomes: Exhibit a more stringent codon recognition, ensuring accurate protein synthesis.
Structural Adaptations
The differences in size and complexity between prokaryotic and eukaryotic ribosomes have functional implications. For instance, the larger size of eukaryotic ribosomes allows for the presence of additional proteins that assist in tRNA binding and codon recognition, contributing to the increased accuracy of protein synthesis.
Moreover, the compartmentalization of eukaryotic ribosomes on the ER enables the efficient targeting of secreted or membrane-bound proteins to their designated locations within the cell. These structural adaptations highlight the specialized roles that prokaryotic and eukaryotic ribosomes play in their respective cellular contexts.
Impact on tRNA Binding and Codon Recognition
The intricate structure of ribosomes governs the precise binding of tRNAs and the recognition of codons during translation. Prokaryotic and eukaryotic ribosomes exhibit distinct structural features that significantly influence these processes.
In prokaryotes, the small subunit (30S) has a 16S ribosomal RNA (rRNA) component and a binding site for tRNAs. The mRNA binds to the 30S subunit, and the Shine-Dalgarno sequence on the mRNA interacts with a complementary sequence on the 16S rRNA, facilitating the correct positioning of the ribosome on the mRNA.
In eukaryotes, the small subunit (40S) has an 18S rRNA component and multiple binding sites for tRNAs. The eukaryotic ribosome is more complex than its prokaryotic counterpart, with additional proteins and RNA components that contribute to its structure and function. The larger eukaryotic ribosome more precisely aligns tRNAs with codons on the mRNA, ensuring accurate codon recognition and translational fidelity.
The differences in ribosome structure impact codon recognition. The decoding center of the ribosome, where codon recognition takes place, is composed of the 16S/18S rRNA and associated proteins. The decoding center in eukaryotic ribosomes is more sophisticated than in prokaryotic ribosomes, allowing for more precise codon recognition and the accommodation of certain variations in codon-anticodon pairings. These structural differences contribute to the higher fidelity of translation in eukaryotes compared to prokaryotes.
Relationship to codon-anticodon pairing and tRNA structure
Relationship to Codon-Anticodon Pairing and tRNA Structure
The codon-anticodon interaction is crucial for the accuracy and efficiency of translation. Codons are three-nucleotide sequences on mRNA that specify which amino acid should be added to the growing protein chain. Anticodons are complementary three-nucleotide sequences on tRNA molecules that bind to codons. This specific binding ensures that the correct amino acid is incorporated into the protein.
tRNA structure plays a vital role in codon-anticodon pairing. Each tRNA molecule has an anticodon loop at one end, which contains the anticodon sequence. The shape of the tRNA molecule allows it to bind to the ribosome in a specific orientation, bringing the anticodon loop into close proximity with the codon on the mRNA.
The precise fit between the codon and anticodon is facilitated by the unique structure of tRNA. The nucleotides in the anticodon loop are highly conserved, ensuring that each codon has a specific tRNA partner. This conservation ensures the fidelity of translation, minimizing errors that could lead to misfolded or non-functional proteins.
Antibiotics targeting translation can disrupt codon-anticodon pairing by interfering with the structure or function of tRNA. For example, the antibiotic streptomycin binds to the 16S rRNA in prokaryotic ribosomes, causing misreading of codons and leading to the incorporation of incorrect amino acids. Conversely, the antibiotic paromomycin binds to the anticodon loop of eukaryotic tRNA, inhibiting its ability to bind to the ribosome and preventing codon recognition.
Understanding the relationship between codon-anticodon pairing and tRNA structure is essential for appreciating the intricacies of protein synthesis and the mechanisms of antibiotics that target this process.
Unique features of prokaryotic and eukaryotic tRNAs
Unique Features of Prokaryotic and Eukaryotic tRNAs
In the intricate ballet of translation, the humble tRNA (transfer RNA) molecule plays a pivotal role, carrying amino acids to the ribosome to assemble the proteins that orchestrate life. But not all tRNAs are created equal. Prokaryotic and eukaryotic tRNAs possess distinct characteristics that reflect the evolutionary divergence between these two kingdoms of life.
Structural Variations:
Prokaryotic tRNAs typically consist of 76 nucleotides, while eukaryotic tRNAs are slightly larger, with around 80 nucleotides. This difference stems from the presence of an extra loop in eukaryotic tRNAs, known as the D loop. The D loop participates in interactions with elongation factors, which are proteins that facilitate the translocation of the ribosome.
Anticodon Modifications:
The anticodon is the region of the tRNA molecule that recognizes and pairs with the complementary codon on the mRNA. In prokaryotes, the first base of the anticodon is often modified, enhancing the ability of the tRNA to decode specific codons. In contrast, eukaryotic tRNAs have a more uniform anticodon structure, with fewer modifications.
Introns and Exons:
Eukaryotic tRNAs often contain introns, non-coding sequences that are removed during RNA processing. This feature is absent in prokaryotic tRNAs. The presence of introns allows for alternative splicing, a mechanism that generates multiple tRNA isoforms from a single gene.
Post-Transcriptional Modifications:
Eukaryotic tRNAs undergo extensive post-transcriptional modifications, including methylation, pseudouridylation, and aminoacylation. These modifications fine-tune the tRNA’s structure and function, enhancing its efficiency in decoding the genetic code.
Recognition by Ribosomes:
The structural differences between prokaryotic and eukaryotic tRNAs influence their recognition by the ribosome. Prokaryotic ribosomes have a simpler structure with fewer protein components, facilitating the binding of tRNAs with modified anticodons. Eukaryotic ribosomes, on the other hand, have a more complex structure and require tRNAs with specific modifications for efficient recognition.
These subtle yet significant differences in tRNA structure and function contribute to the overall complexity of translation in prokaryotic and eukaryotic cells. Understanding these variations is crucial for comprehending the molecular mechanisms that govern protein synthesis and the development of antibiotics that target translation.
Relationship to codon-anticodon pairing
Relationship to Codon-Anticodon Pairing
The codon-anticodon interaction forms the cornerstone of the translation process. Codon sequences, encoded within messenger RNA (mRNA), dictate the sequence of amino acids in a protein, while complementary anticodon sequences on transfer RNA (tRNA) molecules carry the cognate amino acids to the ribosome.
During translation, the ribosome scans the mRNA, pairing codons with complementary anticodons on tRNAs. Codon-anticodon* pairing follows specific rules, ensuring the correct incorporation of amino acids into the growing polypeptide chain. These rules are mostly **universal, shared across all living organisms, which is crucial for the accuracy and efficiency of protein synthesis.
However, some exceptions to universality exist. In some organisms, such as yeast, certain codons can be “read” by multiple tRNAs, known as wobble base pairing. This allows for flexibility in tRNA usage and enables the efficient production of proteins in certain contexts.
Moreover, the structure of tRNAs is intricately tailored to facilitate codon-anticodon pairing. The anticodon loop, a specific region of the tRNA molecule, has a unique shape that promotes optimal interactions with complementary codons. This structural complementarity ensures the precise recognition and binding of tRNA molecules to the ribosome, enabling the accurate decoding of the mRNA sequence.
tRNA Recognition by Ribosomes
In the intricate dance of protein synthesis, where genetic information encoded in DNA is translated into functional proteins, transfer RNAs (tRNAs) play a crucial role as messengers. These tiny molecules, each bearing a specific anticodon sequence complementary to a corresponding codon on the messenger RNA (mRNA), guide the proper incorporation of amino acids into the growing polypeptide chain.
The ribosome, a complex molecular machine, serves as the platform where this meticulous process takes place. Composed of two subunits, the large and the small, the ribosome contains multiple binding sites for tRNAs, each with a specific role.
When a tRNA encounters an mRNA codon, it forms a base-pairing interaction between its anticodon and the codon. This recognition event is facilitated by the ribosome, which monitors the correct matching of the tRNA anticodon to the mRNA codon.
The ribosome, through its specific architecture, provides a precise docking site for tRNA binding. Structural features, such as the anti-codon binding site within the small subunit and the peptidyl-transferase center within the large subunit, ensure that only correctly paired tRNAs are accommodated.
Once a tRNA is bound to the ribosome, it undergoes a conformational change to dock into the ribosomal decoding center. The ribosome then evaluates the pairing of the tRNA anticodon with the mRNA codon. If the pairing is accurate, the ribosome will undergo a series of conformational changes that facilitate the transfer of the amino acid from the tRNA to the growing polypeptide chain.
This intricate process of tRNA recognition by the ribosome is essential for the faithful translation of genetic information. The ribosome, with its sophisticated mechanisms, ensures the precise incorporation of amino acids into the protein, allowing for the creation of functional proteins that play vital roles in all living organisms.
Universality and exceptions in codon-anticodon pairing
Universality and Exceptions in Codon-Anticodon Base Pairing
In the intricate dance of translation, where genetic instructions are turned into functional proteins, the precise pairing of codons and anticodons plays a vital role. While the basic rules of base pairing are largely universal, there are intriguing exceptions that add complexity and fascination to this fundamental process.
Universal Codon-Anticodon Pairing:
The vast majority of codons on messenger RNA (mRNA) are recognized by specific anticodons on transfer RNA (tRNA) molecules. This recognition, governed by the genetic code, ensures that the correct amino acids are incorporated into the growing polypeptide chain. For example, the mRNA codon AUG always pairs with the tRNA anticodon UAC, specifying the amino acid methionine.
Exceptions to the Rule:
However, not all codons strictly adhere to this pairing. In prokaryotes, the rare UGG codon can occasionally code for tryptophan instead of its usual assignment to glycine. This occurs due to a phenomenon called “wobbling,” where the first nucleotide of the anticodon can recognize multiple codons.
In eukaryotes, a more extensive set of exceptions exists. For instance, the CUG codon, which normally codes for leucine, can also specify serine in certain contexts. This flexibility in codon recognition allows for the expansion of the genetic code and the efficient utilization of tRNA pools.
Implications for Protein Synthesis and Genetic Code:
These exceptions to codon-anticodon pairing have profound implications for protein synthesis and the genetic code. They contribute to the redundancy of the genetic code, allowing for multiple codons to specify the same amino acid. This redundancy provides a level of resilience against mutations and ensures the successful translation of essential proteins.
Furthermore, codon recognition exceptions can also influence the efficiency and accuracy of protein synthesis. For example, the ability of some codons to have multiple anticodon matches can lead to competition between tRNAs, potentially slowing down translation and reducing fidelity.
The universality of codon-anticodon pairing is a testament to the fundamental importance of this process in the translation of genetic information. However, the existence of exceptions to the rule adds a fascinating twist to this process and highlights the incredible complexity of biological systems. By delving into these exceptions, we gain deeper insights into the mechanisms that govern protein synthesis and the evolution of the genetic code.
Role of Codon-Anticodon Pairing in tRNA Recognition and Protein Synthesis
In the intricate symphony of protein synthesis, the interplay between codons and anticodons is a crucial dance, ensuring the fidelity and accuracy of protein sequences. Codons, the three-letter nucleotide sequences within mRNA, serve as the molecular blueprints that dictate the order of amino acids in a protein. Anticodons, their complementary partners on transfer RNAs (tRNAs), recognize and bind to specific codons, carrying the appropriate amino acids to the growing polypeptide chain.
This remarkable codon-anticodon pairing process provides the specificity required for accurate protein synthesis. Each tRNA is specialized to recognize a particular codon, ensuring that the correct amino acid is incorporated into the protein. This specificity is essential for the proper functioning of proteins, which rely on the precise arrangement of amino acids to carry out their specific roles within the cell.
The recognition of codons by anticodons is facilitated by the ribosome, the molecular machine that orchestrates protein synthesis. The ribosome has specific binding sites for both mRNA and tRNA, and it carefully monitors the codon-anticodon interactions to ensure that only the correct amino acids are incorporated into the protein.
Any errors in codon-anticodon pairing can lead to incorrect amino acids being incorporated into the protein, potentially disrupting its structure and function. Fortunately, the ribosome has built-in proofreading mechanisms that help to minimize these errors, ensuring the accuracy of protein synthesis.
The importance of codon-anticodon pairing extends beyond protein synthesis. This intricate mechanism serves as a fundamental checkpoint for ensuring the fidelity of genetic information. By meticulously recognizing and pairing the correct codons and anticodons, the cell maintains the integrity of its genetic code, preserving the blueprints for life’s essential proteins.
Codon-Anticodon Base Pairing Rules: The Genetic Code’s Invisible String
The genetic code, the blueprint for life, is a fascinating symphony of nucleotides that determine the sequence of amino acids in proteins. tRNAs, the molecular messengers, carry anticodons that are complementary to the genetic code’s codons. This match-making dance is crucial for accurate protein synthesis.
Prokaryotes and eukaryotes share a universal genetic code, ensuring that genetic information can be translated across these diverse domains. However, there are exceptions that add intrigue to the story. Some codons can be read by multiple anticodons, a phenomenon known as wobble. This allows for flexibility in tRNA recognition, ensuring that the genetic code can be optimally utilized.
The genetic code and tRNA structure are intertwined partners. tRNA molecules have specific structures that facilitate codon-anticodon pairing. The anticodon loop is responsible for recognizing and binding to codons, while the TΨC loop helps stabilize the interaction. These tRNA structural elements ensure that the genetic code is accurately translated into the proteins that form the building blocks of life.
Remember: The relationship between the genetic code, tRNA structure, and codon-anticodon pairing is a testament to the intricate molecular machinery that underpins the very essence of life. It’s a dance where every step is precisely choreographed to ensure the faithful transmission of genetic information.
Mechanism of Action of Elongation Factors: Orchestrating the Dance of tRNA
Elongation factors, the unsung heroes of translation, play a pivotal role in the seamless synthesis of proteins. These molecular maestros work in tandem to facilitate the elongation phase of translation, ensuring the accurate pairing of tRNAs with their corresponding codons on the mRNA.
Step 1: tRNA Delivery
Elongation factors EF-Tu (prokaryotes) and eEF1A (eukaryotes) are the gatekeepers of tRNA delivery. They bind to guanosine triphosphate (GTP), the energy currency of the cell, and form a complex with the appropriate aminoacyl-tRNA. This complex navigates the ribosome, scouting for a vacant A-site.
Step 2: Codon Recognition
The anticodon of the incoming tRNA pairs with the codon in the A-site. The ribosome, acting as a chaperone, stabilizes this pairing, ensuring that the correct amino acid is added to the growing polypeptide chain.
Step 3: Transpeptidation
Elongation factors EF-G (prokaryotes) and eEF2 (eukaryotes) orchestrate the transfer of the amino acid from the tRNA in the A-site to the tRNA in the P-site. This transpeptidation reaction forms a peptide bond, extending the polypeptide chain.
Step 4: tRNA Translocation
The completed tRNA in the P-site is evicted, and the tRNA in the A-site is translocated to the P-site. This movement creates a vacant A-site, ready to receive the next aminoacyl-tRNA.
The cycle of tRNA delivery, codon recognition, transpeptidation, and translocation repeats until a stop codon is encountered, signaling the end of polypeptide synthesis. Elongation factors, the tireless couriers in this molecular symphony, ensure the precise and efficient translation of genetic information into the proteins essential for life.
Differences between Prokaryotic and Eukaryotic Elongation Factors
Elongation factors play a pivotal role in the translation process, facilitating the movement of ribosomes along the messenger RNA (mRNA) and the addition of amino acids to the growing polypeptide chain. Prokaryotes and eukaryotes employ distinct elongation factors with specific characteristics and mechanisms of action.
In prokaryotes, elongation is guided by three main elongation factors: EF-Tu, EF-Ts, and EF-G. EF-Tu is responsible for binding aminoacyl-tRNAs to the ribosome, while EF-Ts promotes the release of EF-Tu from the ribosome. EF-G, on the other hand, drives the translocation of the ribosome along the mRNA, facilitating the movement of the newly added amino acid into the polypeptide chain.
Eukaryotic elongation factors, by contrast, are more numerous and complex. EF-1α, EF-1β, EF-2, and eEF-1A are the key players involved in the elongation process in eukaryotes. EF-1α binds aminoacyl-tRNAs and delivers them to the ribosome, while EF-1β promotes the release of EF-1α. EF-2, similar to its prokaryotic counterpart EF-G, facilitates the translocation of the ribosome. eEF-1A plays a regulatory role, preventing untimely translocation and maintaining the fidelity of the elongation process.
The differences between prokaryotic and eukaryotic elongation factors extend beyond their number and complexity. Prokaryotic elongation factors are typically smaller and simpler in structure compared to their eukaryotic counterparts. Additionally, eukaryotic elongation factors exhibit a higher level of regulation, involving a wider array of protein interactions and modifications.
These distinctions between prokaryotic and eukaryotic elongation factors have implications for antibiotic susceptibility. Antibiotics that target prokaryotic elongation factors, such as erythromycin, are typically not effective against eukaryotes due to their inability to bind the structurally distinct eukaryotic elongation factors.
Relationship to Ribosome Structure, tRNA Structure, and Codon-Anticodon Pairing
The interplay between ribosomes, transfer RNAs (tRNAs), and codon-anticodon pairing forms the foundation of accurate protein synthesis.
- Ribosome Structure: Ribosomes, the molecular machines responsible for translation, exhibit distinct structural features in prokaryotes and eukaryotes. These differences influence the binding of tRNAs. For example, the small subunit of the prokaryotic ribosome contains the 30S ribosomal protein S1, which plays a crucial role in tRNA recognition. Conversely, the large subunit of eukaryotic ribosomes possesses a distinctive peptidyl transferase center, where the nascent polypeptide chain is elongated.
- tRNA Structure: tRNAs, the adapters that deliver amino acids to the ribosome, also display variations in structure between prokaryotes and eukaryotes. Prokaryotic tRNAs are simpler in structure and lack certain modified nucleotides present in eukaryotic tRNAs. These modifications, found in the anticodon loop, enhance codon recognition and optimize tRNA-ribosome interactions.
- Codon-Anticodon Pairing: Codon-anticodon pairing, the interaction between the three-nucleotide codon on mRNA and the complementary anticodon on tRNA, dictates the correct incorporation of amino acids into the growing polypeptide chain. This highly specific pairing ensures the fidelity of protein synthesis. Ribosome structure plays a pivotal role in facilitating codon-anticodon interactions. The ribosomal decoding center, a structural component of the ribosome, provides a stringent environment for codon recognition, maximizing the accuracy of translation.
The Crucial Role of Termination Factors in Translation: Ensuring Accurate Protein Synthesis
At the heart of protein synthesis, the final stage of translation, is the precise and timely termination of the growing polypeptide chain. This critical task is carried out by termination factors, which signal the ribosome to halt the process once the correct protein sequence has been synthesized.
How Termination Factors Orchestrate the Halt
Termination factors recognize stop codons, special sequences in the messenger RNA (mRNA) that signal the end of a protein-coding region. Upon encountering a stop codon, the termination factor binds to the ribosome, causing a conformational change that destabilizes the ribosome-tRNA complex. This disruption releases the newly synthesized protein and cleaves the mRNA from the ribosome, marking the completion of the translation process.
Prokaryotic vs. Eukaryotic Termination Factors
In the world of cells, there are two main domains: prokaryotes and eukaryotes. These domains differ in the composition and function of their termination factors.
- Prokaryotes: Prokaryotic termination factors are a simple duo, known as RF1 and RF2. RF1 recognizes stop codons, while RF2 facilitates the release of the polypeptide chain.
- Eukaryotes: Eukaryotic cells, being more complex, employ a trio of termination factors collectively called eRF1, eRF2, and eRF3. eRF1 recognizes stop codons, eRF2 cleaves the mRNA-ribosome complex, and eRF3 assists in the release of the polypeptide chain.
Relationship with Ribosome Structure and tRNA
The termination factors interact with the ribosome and specific transfer RNA (tRNA) molecules. The ribosome provides the framework for the termination factors to bind and carry out their functions. tRNA molecules, with their anticodons complementary to stop codons, play a crucial role in signaling the termination process.
Importance of Termination Factors
Accurate termination of translation is essential for several reasons:
- Prevents Errors: It ensures the correct length and sequence of synthesized proteins, minimizing the chances of defective or non-functional proteins.
- Genome Integrity: Error-free translation safeguards the genetic information encoded in DNA, preventing mutations that could alter protein structure and function.
- Antibiotic Susceptibility: Differences in termination factors between prokaryotes and eukaryotes make certain antibiotics effective against bacterial infections without harming human cells.
The Intriguing Differences Between Prokaryotic and Eukaryotic Termination Factors
In the realm of protein synthesis, a crucial process that dictates the very essence of life, lies a captivating dance between prokaryotes and eukaryotes. These enigmatic cellular entities, seemingly worlds apart, exhibit striking differences in the way they terminate translation, the final act of this molecular symphony. Termination factors, the maestros of this grand finale, play a pivotal role in ensuring the fidelity and efficiency of protein production.
Prokaryotes, our simpler, ancient brethren, employ a minimalist approach to translation. Their termination factor, aptly named RF2, is a single, self-sufficient protein. This versatile molecule acts as a molecular gatekeeper, recognizing and binding to specific termination codons, the silent cues that signal the end of the translational journey. Upon binding, RF2 triggers a cascade of events, leading to the release of the newly synthesized protein, the dissociation of the ribosome, and the recycling of tRNA molecules.
In contrast, eukaryotes, the more complex and sophisticated of the cellular two, utilize a more elaborate ensemble of termination factors. These eukaryotes possess multiple termination factors, each with specialized roles and target sequences. eRF1, the eukaryotic counterpart of RF2, is responsible for recognizing termination codons. However, its action requires the cooperative effort of eRF3, a protein that binds to eRF1 and stabilizes its association with the ribosome. Together, eRF1 and eRF3 initiate the dismantling process, recruiting GTPase.
GTPase, the molecular engine of the eukaryotic termination machinery, hydrolyzes GTP, providing the energy necessary to break the ribosome apart. This coordinated action between eRF1, eRF3, and GTPase ensures a precise and efficient termination of translation in eukaryotes.
The divergence in termination factors between prokaryotes and eukaryotes reflects their distinct lifestyles and cellular complexities. Prokaryotes, with their rapid growth rates and streamlined cellular machinery, require a more streamlined termination process. Eukaryotes, on the other hand, with their intricate cellular organization and diverse protein repertoire, necessitate a more complex termination system to ensure accurate and efficient protein production.
Relationship to Ribosome Structure, tRNA Structure, and Codon-Anticodon Pairing
In the intricate dance of translation, the ribosome serves as a molecular machine, orchestrating the precise synthesis of proteins. Its structure, a intricate symphony of RNA and proteins, plays a crucial role in this process. The ribosome’s subunits, the small subunit (SSU) and the large subunit (LSU), come together to form a ribosome complex.
Prokaryotic ribosomes, found in bacteria and archaea, are smaller and simpler than their eukaryotic counterparts. They have a 70S sedimentation coefficient, a measure of their size and shape. The prokaryotic SSU binds to the Shine-Dalgarno sequence, a stretch of nucleotides on the mRNA that helps initiate translation.
Eukaryotic ribosomes, present in eukaryotes such as plants, animals, and humans, have an 80S sedimentation coefficient. They have a more complex structure than prokaryotic ribosomes, including additional proteins and RNA molecules. The eukaryotic SSU recognizes the 5′ cap on the mRNA, which aids in translation initiation.
The interaction between the ribosome and tRNA is essential for accurate decoding of the genetic code. The ribosome provides a binding site for tRNA molecules, which carry amino acids to the ribosome complex. The ribosome precisely aligns the tRNA molecules with the mRNA, allowing for base pairing between the anticodon on the tRNA and the codon on the mRNA.
The ribosome structure ensures that the codon-anticodon pairing is accurate and specific. The decoding center, a region of the ribosome, ensures that only the correct tRNA molecule can bind to the ribosome at a given time. This intricate molecular choreography ensures that the genetic information encoded in the mRNA is accurately translated into a protein sequence.
Overview of antibiotics that target different stages of translation
8. Antibiotics Targeting Translation: A Medical Odyssey
Translation, the process of decoding genetic information into proteins, is a vital cog in the cellular machinery of both prokaryotes (bacteria) and eukaryotes (organisms with a membrane-bound nucleus). While the basic principles of translation remain the same, there are subtle yet significant differences between these two domains. These differences have important implications in the medical field, as they make prokaryotic and eukaryotic ribosomes and translation factors prime targets for antibiotics.
The Battleground: Antibiotics vs. Translation
Antibiotics are chemical compounds that target specific cellular processes. Some antibiotics, such as chloramphenicol, erythromycin, and tetracycline, have found their calling in disrupting translation. They do this by binding to different components of the translation machinery, such as the ribosome or initiation factors, interfering with their function.
Prokaryotic vs. Eukaryotic Targets
The differences between prokaryotic and eukaryotic translation machinery translate into specific antibiotics that target one but not the other. For example, aminoglycosides are antibiotics that bind to the 16S rRNA of the prokaryotic ribosome, impairing its ability to bind to tRNA. On the other hand, macrolides like erythromycin specifically target the eukaryotic ribosome, inhibiting its ability to elongate the growing polypeptide chain.
Disrupting the Translation Process
Antibiotics can target various stages of translation. Chloramphenicol, for instance, blocks the peptidyltransferase activity of the ribosome, preventing the formation of new peptide bonds. Erythromycin hinders the translocation of the ribosome along the mRNA, essentially causing a traffic jam in protein synthesis.
Consequences for Protein Synthesis
By disrupting translation, antibiotics ultimately inhibit protein synthesis. This can have profound implications for bacteria, as they depend on a steady supply of proteins for growth, metabolism, and survival. In eukaryotes, antibiotics can potentially affect cell growth, differentiation, and overall cellular function.
The differences between prokaryotic and eukaryotic translation machinery provide a unique opportunity for targeted antibiotic development. By exploiting these variations, scientists can design antibiotics that specifically inhibit bacterial ribosomes or translation factors, leaving eukaryotic cells unscathed. This selectivity is crucial in developing effective antibacterial treatments without harming the host. Understanding the intricacies of translation and the mechanisms of antibiotics that target this process is essential for the ongoing battle against infectious diseases.
Mechanisms of action of antibiotics that target prokaryotic and eukaryotic translation factors
Antibiotics Targeting Translation: Disrupting Protein Synthesis
In the realm of microbiology, the battle between bacteria and antibiotics continues unabated. One critical target of antibiotics is the translation process, the intricate mechanism that converts genetic information into proteins. Let’s delve into how antibiotics exploit differences between prokaryotic and eukaryotic translation to inhibit bacterial growth.
Prokaryotic and Eukaryotic Translation: Unveiling the Differences
The translation process in prokaryotes (bacteria) differs markedly from its eukaryotic counterpart, found in plants, animals, and fungi. These disparities provide unique opportunities for antibiotics to target bacterial translation without affecting host cells.
Antibiotics Targeting Prokaryotic Translation
Aminoglycosides: These antibiotics bind to the bacterial ribosome, interfering with the accuracy of codon-anticodon recognition. This results in the incorporation of incorrect amino acids into the growing polypeptide chain, leading to non-functional or toxic proteins.
Tetracyclines: Tetracyclines also bind to the bacterial ribosome, but they target a different site. They inhibit the binding of charged tRNA molecules to the ribosome, preventing the addition of amino acids to the growing polypeptide chain.
Macrolides: Macrolides, such as erythromycin, bind to the bacterial ribosome and inhibit peptidyl transferase, the enzyme that catalyzes the formation of peptide bonds between amino acids. This blocks the elongation of the polypeptide chain, halting protein synthesis.
Antibiotics Targeting Eukaryotic Translation
While most antibiotics target prokaryotic translation, a few have been developed to specifically target eukaryotic cells.
Cycloheximide: Cycloheximide binds to the eukaryotic ribosome and inhibits protein synthesis by blocking the binding of tRNA molecules to the ribosome. It is primarily used in research settings to study eukaryotic protein synthesis.
Chloramphenicol: Chloramphenicol inhibits bacterial and eukaryotic translation, but it is more potent against bacteria. It binds to the bacterial ribosome and inhibits peptidyl transferase, preventing peptide bond formation and halting protein synthesis.
Importance of Differences in Translation
The differences between prokaryotic and eukaryotic translation are critical for antibiotic susceptibility. By targeting unique features of bacterial translation, antibiotics can effectively combat bacterial infections without harming eukaryotic cells. This specificity allows for the selective killing of bacteria, minimizing side effects and preserving the host’s normal cellular functions.
Antibiotics targeting translation play a crucial role in combating bacterial infections. By exploiting the differences between prokaryotic and eukaryotic translation, these antibiotics selectively inhibit bacterial protein synthesis, hindering their growth and survival. Understanding these differences is essential for the development of new antibiotics and the continued fight against bacterial diseases.
Prokaryotic vs. Eukaryotic Translation: Unraveling the Fundamental Differences
As the blueprints of life, proteins play a pivotal role in shaping the very essence of cells. To create these intricate molecular machines, cells employ a sophisticated process known as translation, where genetic information encoded within mRNA is transformed into a sequence of amino acids. While the fundamental principles of translation remain conserved across all life forms, prokaryotic and eukaryotic cells exhibit distinct variations in this crucial process, mirroring their fundamental differences in cellular architecture and complexity.
Initiation:
The dance of translation begins with the initiation phase, where the ribosome, a molecular machine responsible for protein synthesis, assembles on the mRNA template. In prokaryotes, this assembly is guided by a unique set of initiation factors that orchestrate the binding of the ribosome to a specific start codon, typically AUG. By contrast, eukaryotic initiation involves a more elaborate cast of characters, including additional initiation factors that mediate a complex scanning mechanism to locate the start codon.
Ribosomal Structure:
At the heart of translation lies the ribosome, a two-part structure composed of a small and large subunit. The architecture of these subunits differs significantly between prokaryotes and eukaryotes, influencing both the tRNA binding and codon recognition processes. Prokaryotic ribosomes are smaller and simpler, while eukaryotic ribosomes possess a greater number of proteins and additional subunits, granting them increased complexity and functional versatility.
tRNA Structure and Codon Recognition:
The transfer RNA (tRNA) molecules serve as the translators, carrying amino acids to the ribosome based on the genetic code. Prokaryotic and eukaryotic tRNAs exhibit subtle yet crucial differences in their structure, primarily in the base-pairing interactions within the anticodon loop. These variations directly impact codon recognition, the intricate dance between the codon on the mRNA and the complementary anticodon on the tRNA.
Elongation and Termination:
As the ribosome traverses the mRNA, the process of elongation unfolds, where amino acids are added to the nascent polypeptide chain in a codon-by-codon fashion. Elongation factors facilitate this process, guiding the tRNA-bound amino acids into the ribosome’s catalytic center. Differences in the number and roles of elongation factors between prokaryotes and eukaryotes reflect the varying complexities of their protein synthesis machinery.
The final act of translation is termination, marking the end of protein synthesis. Termination factors orchestrate the release of the newly synthesized polypeptide chain and the disassembly of the ribosome. As with the other stages, termination mechanisms differ between prokaryotes and eukaryotes, highlighting the distinct adaptations that have shaped each branch of the tree of life.
Antibiotics Targeting Translation:
The differences between prokaryotic and eukaryotic translation have profound implications for the development of antibiotics. Many antibiotics exert their бактерицидное действие by targeting specific stages of translation, exploiting the vulnerabilities inherent in the prokaryotic machinery. Understanding these differences provides a crucial foundation for designing antibiotics that effectively combat bacterial infections without harming human cells.
The translation process, a symphony of molecular interactions, showcases the remarkable diversity and adaptability of life on Earth. The differences between prokaryotic and eukaryotic translation reflect the unique evolutionary paths that these two domains of life have taken. By unraveling these intricacies, we gain a deeper understanding of the fundamental mechanisms that drive the synthesis of life’s building blocks—proteins—and pave the way for the development of targeted treatments for a myriad of diseases.
Decoding the Differences: A Tale of Prokaryotic and Eukaryotic Translation
Imagine a microscopic dance, where tiny molecules waltz together to create the protein orchestra that orchestrates life. This intricate choreography is translation, the process of converting genetic blueprints into functional proteins. But did you know that this cellular ballet varies dramatically between two worlds: prokaryotes and eukaryotes?
Initiating the Dance: Initiation Factors
Like the stagehands of a grand production, initiation factors kick off the translation show. These molecular chaperones guide the ribosome, the protein factory, to the correct starting point on the genetic script. Prokaryotic cells rely on a simpler cast of characters, while eukaryotes employ a more elaborate roster. These differences reflect the varying complexities of their genetic machinery.
Ribosome Architecture: A Ballroom Comparison
Prokaryotic and eukaryotic ribosomes, the dance floor for protein synthesis, differ in size, shape, and structural features. These variations impact how tRNA molecules, the carriers of amino acids, bind and interact with the ribosome. It’s like comparing a compact community center to a sprawling cathedral.
tRNA: The Messenger Molecules
Transfer RNA (tRNA) molecules act as message-bearers, delivering amino acids to the ribosome. Prokaryotic and eukaryotic tRNAs wear slightly different outfits, affecting their ability to recognize and deliver the correct amino acids. These variations highlight the importance of tRNA structure in ensuring the accuracy of protein synthesis.
Codon-Anticodon Matchmaking: A Puzzle of Pairs
The genetic code is a set of instructions for protein assembly, and codon-anticodon pairing is the key to deciphering it. Prokaryotes and eukaryotes follow similar pairing rules, but not always strictly. These exceptions reveal the flexibility and robustness of the protein synthesis machinery.
Elongation Factors: Keeping the Rhythm
Elongation factors are the choreographers of the protein-building dance. Prokaryotes and eukaryotes use different dance steps, reflecting the distinct structures of their ribosomes and tRNAs. These variations ensure the smooth flow of amino acid addition, the vital rhythm of protein synthesis.
Termination Factors: Cue for the Curtain Call
When the genetic script reaches its end, termination factors step in to signal the wrap-up of protein synthesis. Prokaryotic and eukaryotic cells use different termination cues, leading to variations in how proteins are released from the ribosome.
Antibiotics: Targeting Translation’s Weaknesses
Antibiotics exploit the differences between prokaryotic and eukaryotic translation to selectively target bacteria. By interfering with key stages of the process, these drugs disrupt protein synthesis and cripple bacterial growth. Understanding these differences is crucial for developing new antibiotics and combating antibiotic resistance.
Prokaryotic and eukaryotic translation are fundamentally different, reflecting the diverse evolutionary paths of these cellular worlds. These differences impact the accuracy, efficiency, and susceptibility to antibiotics of protein synthesis. By grasping these intricacies, we unravel the secrets of life’s molecular dance and gain a deeper understanding of the challenges and opportunities in treating bacterial infections.
Importance of these differences for protein synthesis and antibiotic susceptibility
The Dance of Translation: Prokaryotic vs. Eukaryotic
Translation, the process by which genetic information flows from RNA to protein, is a complex and intricate dance within our cells. Yet, there are subtle but significant differences between how prokaryotes (bacteria and archaea) and eukaryotes (including humans) carry out this fundamental process. Understanding these differences is crucial for protein synthesis and antibiotic susceptibility.
Unveiling the Orchestrators: Initiation Factors
Initiation factors, the conductors of translation, play a pivotal role in starting the protein synthesis process. Prokaryotes use a different set of initiation factors than eukaryotes, reflecting their unique ribosomal structures and tRNA recognition mechanisms. These variations impact the accuracy and efficiency of protein synthesis.
Ribosome’s Structure: A Stage for the Dance
The ribosome, the cellular machinery responsible for protein synthesis, exhibits distinct architectures in prokaryotes and eukaryotes. These differences affect tRNA binding and codon recognition, influencing the precise pairing of amino acids to the genetic code.
tRNA’s Distinctive Roles
Transfer RNAs (tRNAs), the messengers carrying amino acids, have unique features in prokaryotes and eukaryotes. They interact with the ribosome and codon sequences, ensuring correct protein synthesis. Understanding these differences sheds light on tRNA recognition and the maintenance of the genetic code.
Codon-Anticodon: The Language of Protein Construction
Codon-anticodon pairing, the fundamental language of protein synthesis, follows universal rules with some exceptions. These variations influence tRNA recognition and protein structure, highlighting the importance of accurate translation.
Elongation Factors: Fueling the Translation Machine
Elongation factors, the driving force of protein synthesis, facilitate the addition of amino acids to the growing polypeptide chain. Differences between prokaryotic and eukaryotic elongation factors contribute to the varying rates and efficiency of protein production.
Termination Factors: Ending the Show
Termination factors, the stage managers of translation, bring the protein synthesis process to a close. Their distinct mechanisms in prokaryotes and eukaryotes ensure that proteins are produced at the right time and in the right quantities.
Antibiotics: Targeting the Translation Dance
Antibiotics, powerful drugs used to combat infections, often target specific stages of translation. By exploiting the differences between prokaryotic and eukaryotic translation, these drugs can selectively inhibit bacterial protein synthesis without harming human cells. This knowledge has revolutionized medicine, providing effective treatments for bacterial infections.
In conclusion, the differences between prokaryotic and eukaryotic translation are not merely academic curiosities but have profound implications for protein synthesis and antibiotic susceptibility. Understanding these nuances allows us to appreciate the intricate mechanisms that govern the production of life’s essential molecules and opens doors to developing new therapies.