Unraveling The Aufbau Principle: A Guide To Electron Configuration In Atoms
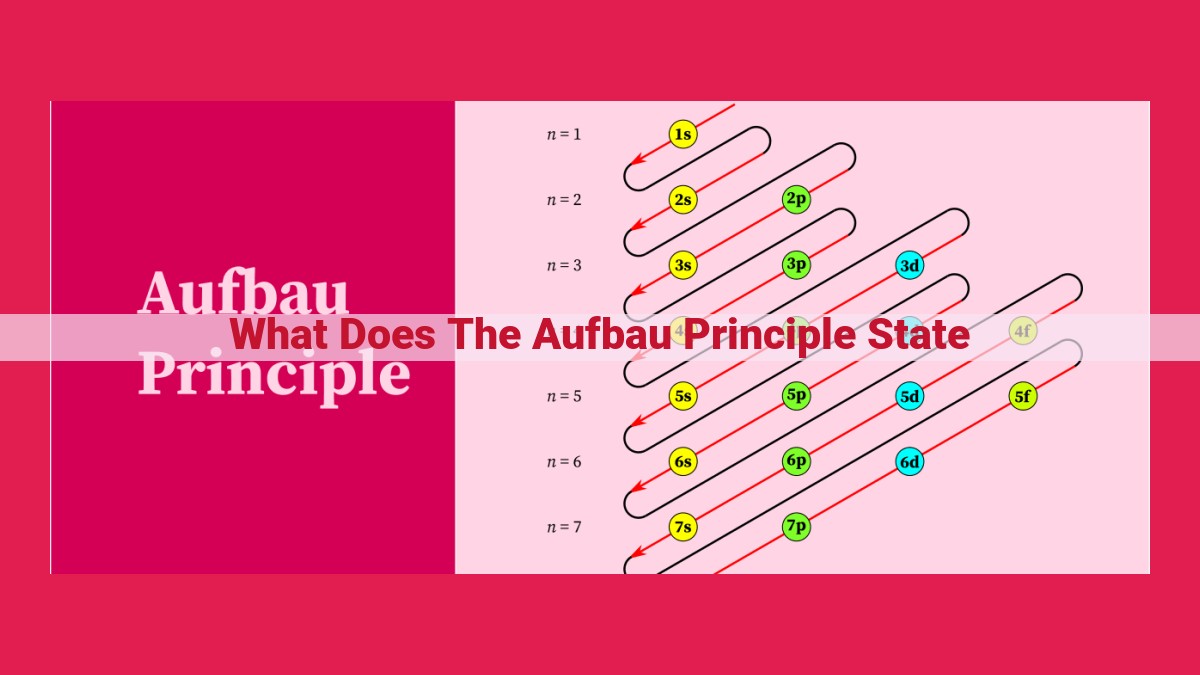
The Aufbau principle, short for Aufbau rule, outlines the order in which electrons fill atomic orbitals, determining the electronic configuration of an atom. It states that electrons occupy orbitals in ascending order of their energy levels, starting with the lowest energy level and working upwards. This principle is crucial for understanding the behavior of electrons in atoms, as it helps predict their arrangement and chemical properties.
The Aufbau Principle: A Guiding Light in the World of Electrons
In the vast expanse of the atomic universe, scientists strive to unravel the enigmatic ways of tiny particles called electrons. One fundamental principle that governs their behavior is known as the Aufbau principle, a guiding light that illuminates the structure of atoms.
The Aufbau principle, also known as the building-up principle, is an essential concept that describes the way electrons arrange themselves within the intricate orbitals surrounding an atom’s nucleus. These orbitals, akin to tiny energy levels, house electrons in a well-defined hierarchy.
Understanding the Aufbau principle is paramount for unraveling the behavior of atoms, the building blocks of all matter. By deciphering the complex dance of electrons, scientists can gain valuable insights into the properties and reactivity of various elements.
The Aufbau principle, with its profound implications, lays the foundation for comprehending the intricate tapestry of the atomic world. Let’s embark on a journey to explore its intricacies and delve into its significance.
Related Concepts
- Atomic orbitals: shape and energy levels
- Electron configuration: arrangement of electrons in orbitals
- Energy levels: quantized units of energy electrons occupy
Understanding the Aufbau Principle: A Journey into Electron Behavior
The Aufbau Principle, a fundamental concept in chemistry, is a guiding force in understanding the intricate behavior of electrons within atoms. This principle dictates the order in which electrons fill atomic orbitals, which are specific regions around the nucleus where electrons are most likely to be found.
Atomic Orbitals: The Electron’s Abode
Imagine atomic orbitals as the electrons’ cozy homes, each with a distinct shape and energy level. These orbitals resemble clouds with varying densities, representing the likelihood of finding an electron at a particular point in space. The shapes of these orbitals are quantized, meaning they can only exist in specific, well-defined forms.
Determining Electron Configuration
The arrangement of electrons in these orbitals is known as electron configuration. Just as a building has different rooms for different purposes, each atomic orbital can accommodate a specific number of electrons. According to the Aufbau Principle, electrons fill the orbitals in order of their increasing energy levels. The lower the energy level, the closer the orbital is to the nucleus, and the more tightly the electrons are held.
Energy Levels: The Quantum Dance
Energy levels are not continuous, but rather exist in quantized steps. Electrons can only occupy specific energy levels, and as they transition from one level to another, they absorb or emit light at specific frequencies. This underlies the colorful glow of neon lights and the distinctive lines in atomic emission spectra.
The Order of Orbital Filling: Unlocking the Secrets of Atomic Structure
The electrons within an atom dance around the nucleus in a mesmerizing ballet, each occupying an orbital—a region of space with a particular energy level. The Aufbau principle orchestrates this dance, guiding electrons to fill orbitals in a specific order.
First and foremost, electrons gravitate toward the lowest energy orbitals. Just like a ball rolling down a hill, electrons seek out the most stable energy states. This minimizes the atom’s overall energy, creating a harmonious equilibrium.
The Pauli exclusion principle adds a mischievous twist to the dance. It decrees that no two electrons can share the same quantum state, like identical twins in different outfits. This rule forces electrons to occupy separate orbitals, ensuring the atom’s stability.
So, how do electrons choose which orbitals to occupy? They follow a hierarchy of energy levels: s, p, d, f. The s orbitals, closest to the nucleus, possess the lowest energy, followed by p, d, and f orbitals.
Within each energy level, electrons fill orbitals in their sublevels. Each sublevel has a specific shape:
- s sublevels: spherical
- p sublevels: dumbbell-shaped
- d sublevels: complex shapes with multiple lobes
- f sublevels: intricate shapes with even more lobes
Electrons first occupy the s sublevels, then the p sublevels, and so on, always filling the sublevel of lowest energy. This hierarchical ordering ensures that atoms achieve their most stable electronic configurations, like puzzle pieces fitting perfectly into place.
The Pauli Exclusion Principle: Unveiling the Coexistence of Electrons
In the enigmatic world of atoms, electrons, the fundamental particles that define their behavior, dance within well-defined energy levels. The Aufbau Principle guides their arrangement, dictating that electrons fill orbitals in ascending order of energy. However, a crucial principle known as the Pauli Exclusion Principle further restricts this cosmic choreography, ensuring that no two electrons can ever occupy the exact same quantum state.
Imagine two electrons as miniature dancers, each vying for a spot on the stage of an atom. According to the Pauli Exclusion Principle, these electrons cannot simply share the same dance floor. Instead, each electron must have its own unique set of quantum numbers, including spin, which can be thought of as the electron’s innate rotational direction. Within each orbital, two electrons can coexist, but only if they possess opposite spins.
This exclusion principle implies that electrons can be likened to tiny magnets, with like poles repulsing each other. If two electrons were to occupy the same orbital with the same spin, their magnetic forces would clash, disrupting the atom’s delicate energy balance. By enforcing this rule, the Pauli Exclusion Principle prevents atomic chaos and ensures that electrons maintain their individuality while harmoniously coexisting within the atom.
The implications of the Pauli Exclusion Principle extend beyond the realm of atoms. It plays a crucial role in determining the chemical properties of elements and shaping the structure of matter as we know it. It explains why certain elements form stable, inert gases while others readily react to form compounds. By understanding the Pauli Exclusion Principle, we gain insight into the intricate dance of electrons that underlies the very fabric of our universe.
Limitations of Orbital Occupancy
As we explore the quantum world of atoms, we encounter the Aufbau Principle, which guides the arrangement of electrons within their orbitals. This principle dictates the order in which electrons fill orbitals, starting with the lowest energy level and progressing upwards. However, there are limitations to this orbital occupancy, governed by two fundamental principles: the Pauli Exclusion Principle and Hund’s Rule.
The Pauli Exclusion Principle
The Pauli Exclusion Principle states that no two electrons can occupy the exact same quantum state within an atom. This means that each orbital can accommodate a maximum of two electrons, and these electrons must have opposite spins. This principle arises from the wave-particle duality of electrons and their anti-symmetrical wave functions.
Hund’s Rule
Hund’s Rule further governs the arrangement of electrons within orbitals. It states that electrons occupy the available orbitals with the same spin before pairing up. This results in the distribution of electrons evenly among the available orbitals, maximizing the atom’s magnetic moment.
Hund’s Rule, named after the German physicist Friedrich Hund, is important for understanding the behavior of unpaired electrons in atoms. It plays a crucial role in determining the magnetic properties of elements and compounds, as unpaired electrons contribute to the material’s overall magnetism.