Atp: The Cellular Energy Currency For Mechanical Work
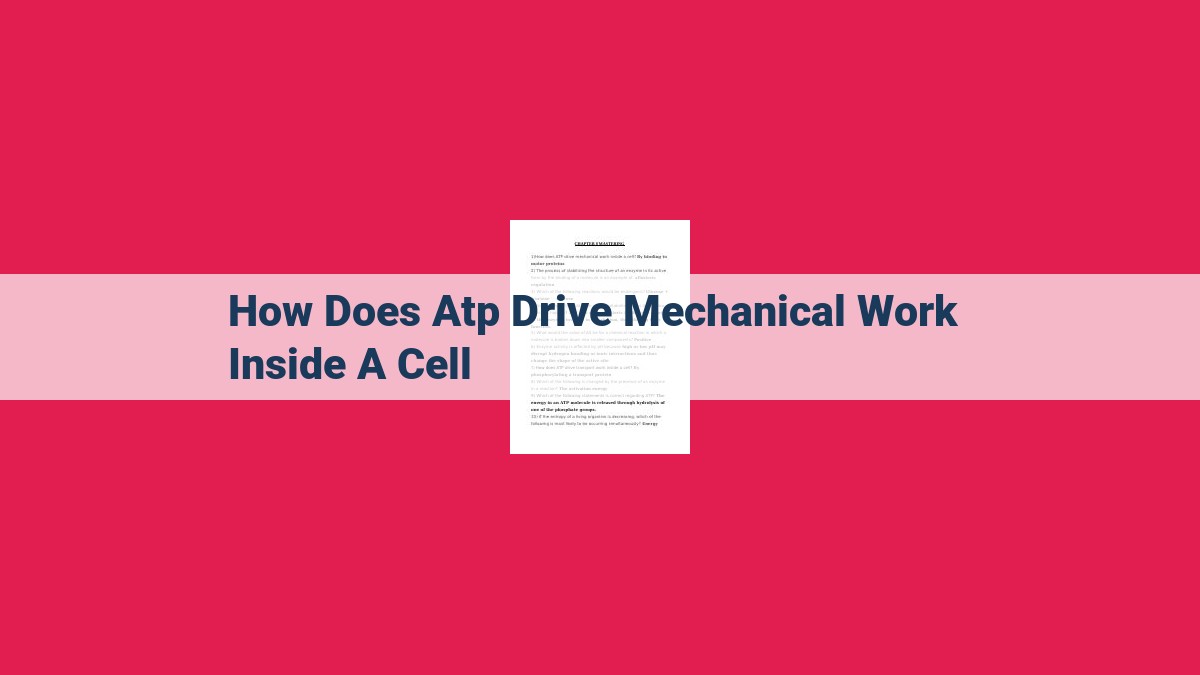
ATP, the cell’s energy currency, drives mechanical work by undergoing hydrolysis, releasing energy that powers conformational changes in motor proteins. These proteins convert the energy into mechanical force, using it for various cellular processes such as muscle contraction, cell division, and organelle transport. Protein-protein interactions and molecular motors link ATP hydrolysis directly to mechanical work, enabling cells to tightly regulate force production and carry out essential functions with precision.
ATP: The Powerhouse that Drives Cellular Machines
Paragraph 1:
Inside the bustling metropolis of a cell, there exists a tiny yet mighty molecule: ATP, the primary energy currency that powers its intricate machinery. Like a bustling power plant, ATP provides the fuel to drive everything from muscle contractions to cell division, enabling life’s essential processes to unfold.
Paragraph 2:
When ATP’s chemical bonds break, they release an energy surge that fuels cellular reactions. The wonders of energy metabolism ensure a steady supply of ATP, giving cells the power they need. Just as gasoline fuels a car, ATP’s energy drives the cell’s molecular machines, propelling them to perform their vital tasks.
Paragraph 3:
Among the cell’s molecular machines, a group known as motor proteins stands out. Motor proteins have the remarkable ability to undergo shape changes, akin to contortionists performing incredible feats. These shape changes are the key to understanding how ATP unleashes mechanical work within cells.
ATP Hydrolysis: Unleashing Energy for Cellular Work
ATP, the ubiquitous energy currency of cells, plays a pivotal role in powering cellular processes. Its remarkable ability to release energy through hydrolysis drives a multitude of mechanical tasks within the cell.
Process of ATP Hydrolysis: Breaking Down the Energy Storehouse
ATP contains a high-energy bond between its adenosine and triphosphate groups. This bond, when broken by hydrolysis, releases the stored energy. The process involves the addition of a water molecule, which splits the bond, resulting in ADP (adenosine diphosphate) and inorganic phosphate (Pi).
Energy Metabolism: Fueling the ATP Cycle
The constant supply of ATP required for cellular work is maintained through a complex network of metabolic pathways. Glycolysis, oxidative phosphorylation, and other energy-generating processes produce ATP and other energy carriers that drive hydrolysis reactions. The breakdown of glucose, fats, and amino acids replenishes the ATP pool, ensuring an uninterrupted supply of energy for cellular activities.
In conclusion, ATP hydrolysis serves as a fundamental mechanism for releasing energy in cells. This process, fueled by cellular metabolism, provides the driving force for a vast array of mechanical tasks, empowering cells to perform vital functions that sustain life.
Conformational Changes in Motor Proteins: The Secret Power Behind ATP’s Mechanical Magic
Motor proteins, the tiny workhorses inside our cells, are the key players in transforming ATP’s energy currency into mechanical force. These molecular machines are capable of undergoing dramatic conformational changes that drive the cellular processes essential for life.
The energy fueling these conformational changes comes from the hydrolysis of ATP, a process that releases energy by breaking its chemical bonds. This energy is captured by motor proteins, which use it to alter their shape and move along cellular tracks.
Think of motor proteins as tiny engines, with their heads binding to cellular tracks and their tails tethered to cargo molecules. The hydrolysis of ATP powers the engine’s combustion, causing the head to pivot like a ratchet, pulling the cargo molecule forward.
Mechanical Force Generation: How ATP Drives Cellular Motion
As we dive deeper into the realm of cellular mechanics, we now uncover the secrets behind how ATP powers the generation of mechanical force within cells. This extraordinary process hinges on the remarkable conformational changes that motor proteins undergo, fueled by the energy harnessed from ATP hydrolysis.
Imagine a microscopic tug-of-war taking place within the cell, with motor proteins playing the role of molecular workhorses. These proteins bind to cytoskeletal filaments, the structural scaffolding of the cell, and, like a molecular ratchet, inch along by undergoing a series of precisely orchestrated conformational changes. Each step forward, powered by the release of ATP energy, generates a tiny but significant amount of force.
The biophysical and mechanical principles governing force production in cells are nothing short of fascinating. As ATP hydrolysis occurs, it triggers conformational changes in motor proteins, causing them to change shape and angle. This seemingly subtle shift in orientation, coupled with the tight binding to cytoskeletal filaments, results in force generation.
The magnitude and direction of the force depend on the specific motor protein involved and the nature of its interaction with the filament. Different motor proteins exhibit specialized functions, tailored to specific forms of mechanical work within the cell. For instance, some motor proteins excel at generating linear force, driving processes like muscle contraction and cell division, while others are adept at generating rotational force, facilitating organelle transport and the twisting of DNA during transcription.
In essence, mechanical force generation in cells is a testament to the wonders of molecular machinery, where ATP hydrolysis fuels the conformational changes in motor proteins, which, in turn, translate into precise and controlled force production. This intricate choreography of molecular interactions is the driving force behind a multitude of cellular processes, ranging from the beating of our hearts to the intricate dance of chromosomes during cell division.
Mechanical Work in Cells: The Vital Force Behind Cellular Processes
ATP’s Role in Driving Cellular Machinery
Cells, the fundamental units of life, are bustling with activity, driven by the constant flow of energy. This energy is primarily harnessed in the form of adenosine triphosphate (ATP), the cellular powerhouse. ATP serves as the universal energy currency of cells, providing the fuel for a multitude of essential processes.
One of the most crucial roles of ATP is to drive mechanical work, providing the energy that powers the intricate machinery within cells. This mechanical work finds applications in a wide range of cellular processes, including muscle contraction, cell division, and the movement of organelles.
Muscle Contraction: A Symphony of Force
Muscles, the tireless engines of our bodies, rely on the power of ATP to generate force and movement. When a nerve impulse triggers a muscle fiber, ATP hydrolysis releases energy that drives the sliding of filaments within the muscle cells. This sliding motion causes the muscles to contract, enabling us to perform a vast array of actions, from running and jumping to grasping objects.
Cell Division: A Delicate Dance of Force
The intricate process of cell division requires precise manipulation of chromosomes. Here, ATP hydrolysis fuels motor proteins that move chromosomes along microtubules, ensuring their proper distribution during cell division. This carefully orchestrated dance of force ensures that each daughter cell receives a complete set of genetic material.
Organelle Movement: A Logistical Masterpiece
Within the crowded confines of cells, organelles must navigate their way to perform their specific tasks. ATP-dependent motor proteins act as cellular Sherpas, transporting organelles along cytoskeletal tracks. This intricate logistics system ensures that organelles reach their destinations, enabling the efficient functioning of the cell.
ATP’s Contribution: A Story of Precision
The ability of ATP to drive mechanical work highlights the remarkable versatility of this molecular powerhouse. Through its precise control of energy release, ATP empowers cells to perform a breathtaking array of tasks. From the thunderous power of muscle contraction to the delicate ballet of cell division, ATP is the unsung hero behind the vital forces that drive our very existence.
ATP-Protein Coupling: The Driving Force Behind Cellular Movement
In the intricate world within our cells, the energy currency known as ATP plays a pivotal role in driving the mechanical processes that power life. ATP’s interactions with proteins, particularly molecular motors, form the cornerstone of this energy-driven machinery.
Molecular motors are fascinating molecular machines that convert the chemical energy of ATP hydrolysis into mechanical force. This force is harnessed to perform various tasks essential for cellular function, such as muscle contraction, cell division, and the transport of cellular cargo.
ATP’s grip on molecular motors is what enables these proteins to execute their mechanical functions. Upon binding to ATP, molecular motors undergo conformational changes, shifting their shape and position. This ATP-dependent conformational change is the driving force behind the generation of mechanical force.
As ATP hydrolysis occurs, the bond between the terminal phosphate group and the adenosine molecule is broken, releasing energy. This energy is channeled into the conformational change of the motor protein, causing it to swing or step forward against a resisting force.
The ATP-protein coupling mechanism allows for precise control of mechanical force production. Cells can finely tune the activity of molecular motors by regulating ATP hydrolysis, ensuring that force is generated only when and where it is needed. This intricate control system is essential for the smooth and coordinated execution of cellular processes.
In conclusion, ATP-protein coupling represents a remarkable molecular mechanism that enables cells to convert chemical energy into mechanical force. This energy-driven machinery is the lifeblood of cellular movement, driving essential processes that sustain life itself.
Motor Protein Diversity: The Specialized Workhorses of Cells
In the bustling metropolis of a cell, there exists a remarkable class of proteins known as motor proteins. These molecular workhorses are responsible for generating the mechanical force necessary for countless cellular processes, from muscle contraction to cell division. But what sets these proteins apart is their incredible diversity, each one tailored to perform a specific task with unmatched efficiency.
Like specialized tools in a toolbox, motor proteins come in various shapes and sizes, each equipped with unique capabilities. Myosin II, for instance, is a muscle giant, its role being the powerful contraction of muscles. Its elongated shape and ability to “walk” along actin filaments, the cell’s structural scaffolding, enable it to generate the force required for movement.
Kinesin, a more delicate motor, excels at transporting cellular cargo along microtubules, the cell’s highway system. Its two globular heads, like tiny feet, take alternating steps, allowing it to glide effortlessly along these tracks, delivering essential molecules to their destinations.
Dynein, another microtubule motor, possesses an impressive pulling power. It’s responsible for transporting large organelles, such as nuclei and mitochondria, around the cell, ensuring they reach their proper locations.
The diversity of motor proteins extends far beyond these examples. Each cell type harbors a unique repertoire of motor proteins, carefully selected to meet its specific functional demands. This remarkable adaptability underscores the intricate symphony that orchestrates cellular life.
ATP Hydrolysis Regulation: Controlling Mechanical Work
In the intricate dance of life’s processes, ATP stands as the cellular powerhouse, fueling myriad functions. Among its roles, ATP drives mechanical work within cells, enabling essential tasks like muscle contraction and cell division. The intricate interplay between ATP hydrolysis and motor protein conformational changes generates the forces that power these processes.
However, this dance is not without its conductors. Signal transduction and cellular regulation wield the baton, orchestrating the precise control of mechanical work. Cellular signals, like calcium ions, act as messengers, carrying instructions that regulate ATP hydrolysis. By fine-tuning the rate of ATP breakdown, cells can tailor the force and duration of mechanical work.
The mechanisms behind this exquisite control involve the interplay of molecular switches and feedback loops. Motor proteins, the molecular engines of mechanical work, are equipped with regulatory domains that respond to cellular signals. These switches can inhibit or enhance ATP hydrolysis, adjusting the protein’s activity accordingly. Feedback loops further refine this control, ensuring that force production is appropriate for the task at hand.
This precise regulation of ATP hydrolysis is essential for the coordinated functioning of cells. Disruptions to these regulatory mechanisms can lead to pathological conditions, such as abnormal muscle contractions or impaired cell division. Understanding the intricate interplay between ATP hydrolysis and regulation is crucial for unraveling the complex tapestry of life’s processes.