Atomic Properties: Key Determinants Of Chemical Behavior
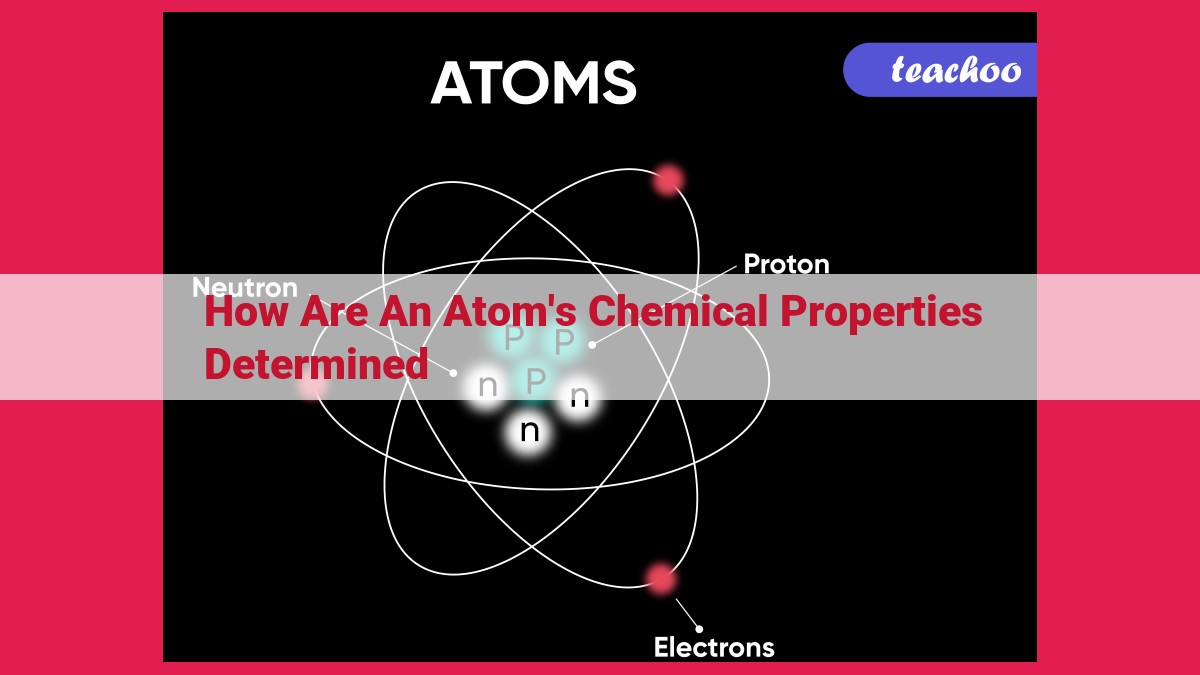
An atom’s chemical properties are determined by its atomic number, electron configuration, and the behavior of its valence electrons. The atomic number defines the number of protons, which in turn determines the number of electrons. The electron configuration describes the arrangement of electrons in orbitals, which influences the atom’s energy and reactivity. Valence electrons are the electrons in the outermost orbital and are responsible for forming chemical bonds. Ionization energy, electron affinity, and electronegativity are measures that quantify an atom’s ability to lose or gain electrons and its attraction for electrons, respectively. These properties collectively determine how an atom interacts with other atoms, forming the basis of its chemical behavior.
Atomic Architecture: The Foundation of Chemical Identity
In the sprawling realm of science, chemistry stands out as a tapestry woven from the intricate interplay of atoms – the elemental building blocks of our universe. To unravel the secrets of chemical identity, embark on a journey into the heart of the atom, where the atomic nucleus reigns supreme.
At the helm lies the atomic number, a cornerstone concept that defines the number of protons within the nucleus. Protons, with their positive charge, establish the core of the atom, determining its distinctive identity. Alongside protons, neutrons, their neutral counterparts, contribute to the atom’s mass without altering its overall charge.
The mass number, a summation of protons and neutrons, provides a glimpse into the atom’s heft. Elements, the fundamental units of chemistry, are classified based on their unique atomic numbers. This atomic architecture serves as the blueprint for chemical diversity, dictating an element’s properties and dictating its behavior when it interacts with its atomic neighbors.
Electron Symphony: The Dance of Electrons in Orbitals
In the captivating world of chemistry, electrons play a pivotal role in shaping the identity of atoms and their remarkable ability to interact. Like a harmonious dance, electrons reside in specific regions around the nucleus, forming the fundamental building blocks of matter. These regions, known as orbitals, are the stage upon which electrons perform their intricate choreography.
Orbital Arrangements: The Quantum Dance
Each electron inhabits a distinct orbital, characterized by a unique set of quantum numbers. The principal quantum number (n) determines the electron’s energy level, while the angular momentum quantum number (l) defines its shape. Picture an electron as a ballerina, gracefully pirouetting within a specified orbital, its every move governed by these quantum numbers.
Shaping the Atomic Symphony
The distribution of electrons across orbitals profoundly affects an atom’s overall energy and chemical behavior. Electrons with higher energy levels occupy orbitals further from the nucleus, while those closer to the nucleus possess lower energy. The arrangement of electrons also determines the atom’s oxidation state and reactivity. Like a conductor leading an orchestra, the distribution of electrons orchestrates the atom’s interactions with other elements.
The Energetic Tango: Ionization and Electron Affinity
The ionization energy of an atom measures the energy required to remove an electron from its outermost orbital. This property serves as a gauge of the atom’s reluctance to part with its electrons. In contrast, electron affinity quantifies the energy released when an electron is added to an atom. This energy gain reflects the atom’s eagerness to acquire electrons. The interplay between ionization energy and electron affinity dictates the atom’s propensity to form ions, influencing its chemical reactions.
Valence Electrons: The Gatekeepers of Reactivity
Imagine the world of atoms as a grand symphony, where each element plays a unique melody. Valence electrons, like the lead singers of this symphony, take center stage in determining the chemical identity and behavior of these atomic performers.
Valence electrons are the electrons in an atom’s outermost energy level. They are the ones that actively participate in chemical bonding, the process by which atoms join hands to form molecules. Like a bridge between atoms, valence electrons share their dance moves to create the tapestry of chemical compounds we see around us.
The number of valence electrons an atom possesses has a profound impact on oxidation states—the formal charge of an atom when it forms bonds. Atoms eagerly give or receive electrons to attain a stable octet of valence electrons, which is the ideal configuration for chemical harmony. This electron give-and-take influences an atom’s reactivity, making them more or less willing to participate in chemical reactions.
Atoms with a high number of valence electrons tend to be electron donors, readily forming negative ions. These elements, like the hospitable host of a party, invite other atoms to join their electron-rich dance. Conversely, atoms with a low number of valence electrons become electron acceptors, seeking to fill their empty electron orbitals. They’re like the eager students in a classroom, always raising their hands, eager to learn and share.
Ionization Energy: The Cost of Electron Liberation
Defining Ionization Energy:
Ionization energy, like the toll of a drawbridge, represents the energy required to detach an electron from an atom or ion. It quantifies the strength with which electrons are held within an atom’s grip.
Factors Influencing Ionization Energy:
Several factors play a role in determining an atom’s ionization energy:
-
Atomic Size:
Electrons in larger atoms experience a weaker pull from the nucleus, making them easier to remove and lowering ionization energy. -
Nuclear Charge:
As the number of protons in the nucleus increases, the attraction for electrons strengthens, leading to higher ionization energy. -
Electron Configuration:
The atomic orbitals in which electrons reside also impact ionization energy. Filled orbitals tend to shield outer electrons from the nucleus, decreasing ionization energy.
Variation Across Elements:
Ionization energy follows a periodic trend:
-
It generally increases from left to right across a period, reflecting the increasing nuclear charge.
-
It decreases down a group, as the number of atomic orbitals increases and shielding becomes more effective.
Relationship to Oxidation States and Electronegativity:
Ionization energy is closely related to oxidation states. Elements with low ionization energies tend to form positive ions easily and thus have high oxidation states.
Electronegativity, which measures an atom’s ability to attract electrons, also correlates with ionization energy. High electronegative elements have high ionization energy because their electrons are held firmly.
Electron Affinity: The Energy of Electron Acquisition
In the captivating world of chemistry, we delve into the intricacies of electron affinity, an enigmatic force that governs an atom’s ability to acquire electrons. This alluring dance of attraction and repulsion plays a pivotal role in shaping the chemical destiny of each element.
Defining Electron Affinity
Electron affinity is the energy change that occurs when a neutral atom gains an extra electron. It’s a measure of the atom’s eagerness to embrace electrons into its embrace. The more negative the electron affinity, the more enticing it is for the atom to attract electrons.
Impact on Reduction Potentials and Electronegativity
Electron affinity holds a profound influence on reduction potentials. Reduction, the process of gaining electrons, becomes more favorable for atoms with higher electron affinities. Additionally, electron affinity correlates closely with electronegativity, the atom’s ability to attract electrons in a chemical bond.
Significance in Negative Ion Formation
A crucial implication of electron affinity lies in determining an atom’s ability to form negative ions. When an atom acquires an additional electron, it transforms into a negative ion. The greater the electron affinity, the easier it is for the atom to form negative ions.
Electronegativity and Electron Affinity
Electron affinity and electronegativity are intimately linked. An atom with a high electron affinity tends to have a high electronegativity, indicating its strong ability to attract electrons. This harmonious relationship orchestrates the formation of stable chemical bonds between atoms.
In the vast tapestry of chemical elements, each exhibits a unique electron affinity, shaping its reactivity, bond formation, and the symphony of chemical reactions that orchestrate the material world around us.
Electronegativity: The Attraction of Electrons
In the realm of chemistry, electronegativity plays a pivotal role in shaping the chemical bonding and molecular interactions. It refers to the ability of an atom to attract electrons towards itself in a chemical bond. This captivating concept unveils the secrets behind the formation of covalent and ionic bonds, providing a deeper understanding of the forces that govern the molecular world.
Electronegativity is closely intertwined with electron affinity and bond polarity. Electron affinity measures the attraction of an atom for an additional electron, while bond polarity indicates the uneven distribution of electrons in a covalent bond. Electronegativity serves as a bridge between these concepts, influencing the extent of both electron affinity and bond polarity.
The greater the electronegativity of an atom, the stronger its pull on electrons. This attraction drives the formation of polar covalent bonds, characterized by an unequal sharing of electrons. The atom with higher electronegativity will possess a partial negative charge, while the atom with lower electronegativity will have a partial positive charge.
The differences in electronegativity determine the type and strength of chemical bonds formed. Large differences lead to the formation of ionic bonds, where one atom completely transfers an electron to the other, creating oppositely charged ions. Smaller differences result in covalent bonds, where electrons are shared between atoms. The strength of a covalent bond is directly proportional to the electronegativity difference between the two atoms involved.
By delving into the captivating tale of electronegativity, we unravel the secrets of chemical bonding and molecular interactions. This fundamental concept illuminates the attractions and repulsions that govern the behavior of atoms, shaping the vast panorama of chemical compounds that grace our world.