Understanding Atomic Collisions: Energy Exchange, Chemical Reactions, And Reaction Paths
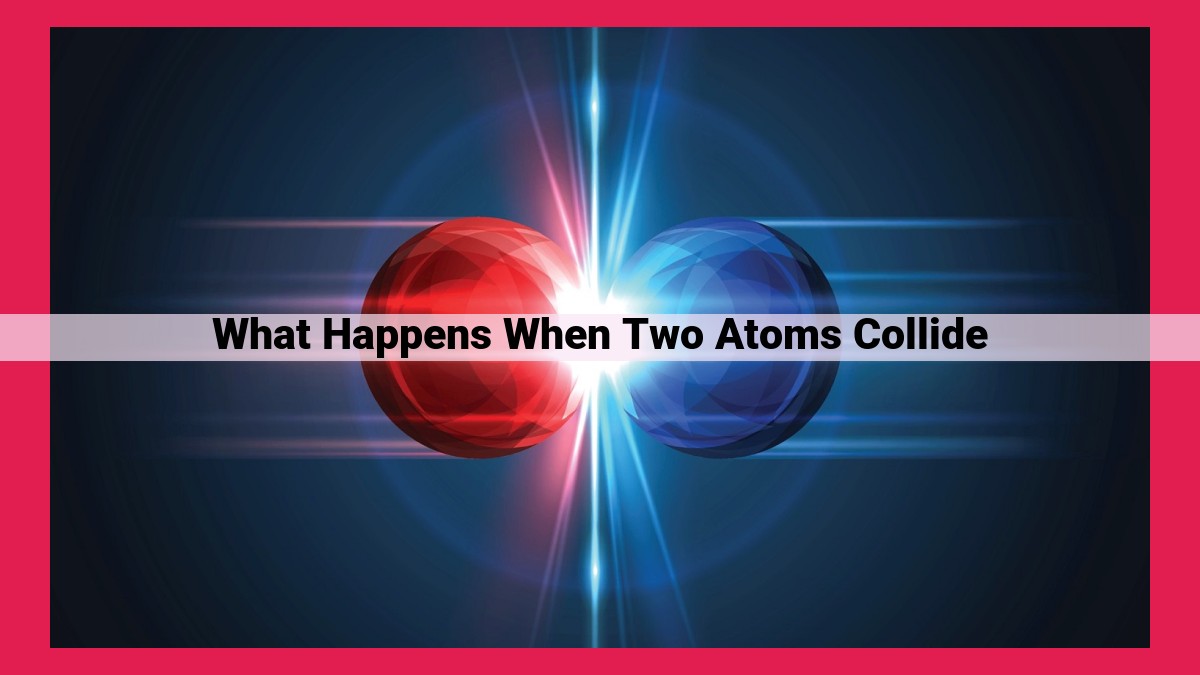
When atoms collide, the outcome depends on factors like their energy and the nature of the collision. Elastic collisions conserve the total energy of the system, with the atoms bouncing off each other with no change in internal energy. Inelastic collisions involve energy transfer, potentially leading to changes in the atoms’ electronic or vibrational states. Exothermic collisions release energy, while endothermic collisions absorb energy. Activation energy is the minimum energy required for a collision to initiate a chemical reaction. The reaction path involves a transition state, a high-energy intermediate species, before the final products are formed.
Delving into the Intriguing World of Collisions: The Physics Unveiled
In the bustling realm of atoms and molecules, collisions play a pivotal role in shaping the dynamics of our universe. From the spectacular explosions of celestial bodies to the subtle interactions within living cells, collisions govern a myriad of phenomena. Let’s embark on a captivating journey to unravel the physics that underpins these fascinating events.
Atomic Collisions: A Dance of Energy and Momentum
Imagine two tiny particles, say, atoms or molecules, hurtling towards each other with a specific amount of energy and momentum. When they collide, their trajectories and energies undergo a dramatic transformation. This encounter is what we refer to as an atomic collision.
Collisions can be broadly classified into two main types: elastic and inelastic. In elastic collisions, the total kinetic energy of the particles involved remains conserved, meaning it remains unchanged. Like billiard balls bouncing off each other, these particles bounce apart with the same combined energy they had before colliding.
In contrast, inelastic collisions are characterized by a transfer of kinetic energy, either into or out of the system. Think of a car crash where the vehicles absorb some of the kinetic energy from the impact, resulting in deformation or even damage. In such collisions, the total kinetic energy decreases or increases, depending on whether the collision is exothermic or endothermic.
Understanding the physics of collisions is crucial for unraveling the mysteries of the universe. From the formation of stars to the reactions that occur within our own bodies, atomic collisions play a fundamental role in shaping the world as we know it.
Energy Exchange in Collisions: Unraveling the Secrets of Exothermic and Endothermic Encounters
In the bustling realm of atomic collisions, energy plays a pivotal role, shaping the outcomes of these microscopic interactions. When atoms or molecules collide, they exchange energy, giving rise to two distinct types of collisions: exothermic and endothermic.
Exothermic Collisions: Energy Released
Imagine two cars crashing head-on. The impact releases a burst of energy in the form of heat, sound, and light. Similarly, in exothermic collisions, the colliding particles release energy as they collide. This energy is often observed as an increase in thermal energy or the emission of electromagnetic radiation.
Endothermic Collisions: Energy Absorbed
In contrast, endothermic collisions are like uphill battles. In these encounters, the colliding particles absorb energy as they come together. This absorbed energy is stored as potential energy within the newly formed molecules or atoms. Endothermic collisions typically require an external energy source, such as heat or light, to initiate the reaction.
Implications for the System’s Energy
The nature of the collision, whether exothermic or endothermic, has a profound impact on the energy of the system involved. In exothermic collisions, the total energy of the system decreases as energy is released. This released energy can be used to power other reactions or processes.
Conversely, in endothermic collisions, the total energy of the system increases as energy is absorbed. This absorbed energy must be provided by an external source, otherwise the reaction will not proceed.
Comprehending the energy exchange in collisions is crucial for deciphering the dynamics of chemical reactions. By unraveling these microscopic interactions, scientists gain insights into the intricate tapestry of atomic and molecular behavior that shapes the world around us.
Reaction Dynamics: The Ignition Point of Chemical Reactions
Every chemical transformation is sparked by a tiny spark of energy, a catalyst that initiates the dance of atoms and molecules leading to the formation of new substances. This spark is known as activation energy, the minimum amount of energy required to break existing bonds and allow new ones to form.
Imagine a group of hikers on a mountain trail. To reach the summit, they must overcome an obstacle course of towering rocks and treacherous slopes. Activation energy is like the barrier they must scale before they can embark on their climb towards a new chemical landscape.
Once this energy hurdle is cleared, the reactants, the hikers in our analogy, begin their journey to become products, the hikers reaching the summit. This path they travel is known as the reaction path. Along this path, a critical point emerges—the transition state, where the reactants are at their most unstable and poised to transform into products.
The transition state is a fleeting moment, like a hiker balancing precariously on a narrow ledge. It represents the highest energy point on the reaction path, the peak the hiker must conquer before descending into the realm of products. Chemical reactions, like mountain treks, are driven by the relentless pursuit of lower energy states, the hikers’ desire to reach the summit.
The Reaction Path and Transition State: Unveiling the Journey of Chemical Transformations
Chemical reactions are not instantaneous events; they involve a series of intricate steps that lead to the transformation of reactants into products. At the heart of this process lies the reaction path, a roadmap that depicts the sequence of events as the reactants progress towards their final destination.
Along this path, molecules collide, exchange energy, and undergo various transformations. A crucial stage in this journey is the transition state, a transient state that marks the highest energy point on the reaction path. Imagine a mountain pass that separates two valleys, representing the reactants and products. The transition state is the summit of this mountain pass, where the molecules possess the maximum energy required to overcome the energy barrier and proceed towards the products.
The formation of the transition state is a dynamic process. Reactant molecules approach each other, their bonds begin to stretch and rearrange, and new bonds start to form. The transition state represents a fleeting moment where the old and new bonds coexist, creating a highly unstable and energized molecular configuration.
Once the transition state is reached, the molecules have two possible fates. They can either descend down the other side of the mountain pass, towards the products, or they can retreat back down the path, towards the reactants. The probability of either outcome depends on the energy available to the molecules and the height of the energy barrier they need to overcome.
If the molecules have sufficient energy to surmount the energy barrier, they will proceed towards the products. Bonds will continue to break and form, and the molecules will eventually reach their final destination as stable products. However, if the molecules do not possess enough energy, they will fall back towards the reactants, and the reaction will not occur.
Understanding the reaction path and transition state is essential for comprehending the mechanisms of chemical transformations. It provides insights into the rates of reactions, the selectivity of reactions, and the activation energies required for reactions to occur. By unraveling the intricacies of the reaction path, we gain a deeper appreciation of the fascinating world of chemical reactivity.