Unlocking The Versatility Of Carbon: Its Atomic Structure And Significance In Organic Chemistry
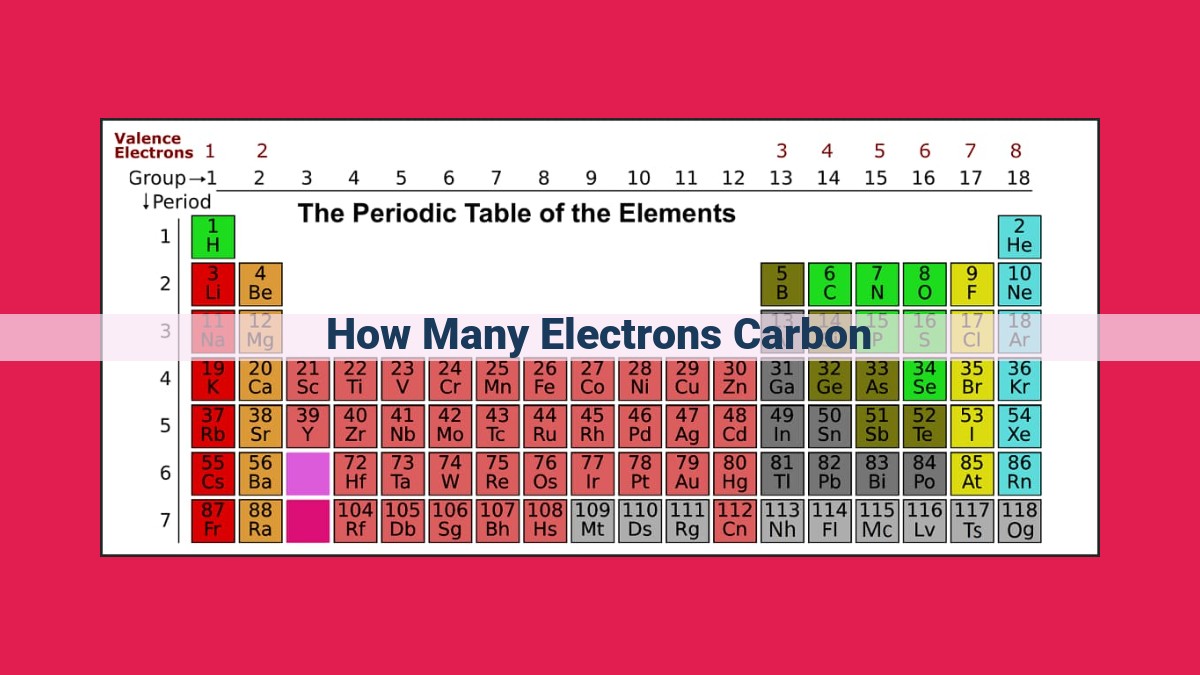
Carbon, the sixth element in the periodic table, holds a unique position due to its atomic structure. It possesses six electrons, with two in the innermost shell and four in the valence shell responsible for chemical bonding. This electron configuration makes carbon highly versatile, allowing it to form covalent bonds with itself and other atoms, creating a vast array of organic molecules essential for life on Earth.
Carbon: The Essential Building Block of Life
- Discuss the different isotopes of carbon and their significance.
- Explain the electron configuration and atomic number of carbon.
Carbon: The Essential Building Block of Life
Carbon, the sixth element on the periodic table, forms the backbone of all living organisms. It is a versatile element with many forms, from the spark of a diamond to the soot on a candle flame.
Isotopes and Atomic Structure
Carbon exists in two stable isotopes: carbon-12 and carbon-14. Carbon-12 is the most abundant and the basis for defining the atomic mass of all other elements. Carbon-14, on the other hand, is radioactive and has a half-life of 5,730 years. Its decay rate is used in carbon dating, a technique to determine the age of organic material.
Carbon’s atomic number is six, meaning it has six electrons orbiting its nucleus. Its electron configuration is 1s²2s²2p², giving it four valence electrons – the electrons available for chemical bonding.
Electrons and Chemical Bonding
Valence electrons dictate an element’s chemical reactivity. Carbon’s four valence electrons allow it to form strong covalent bonds, sharing electrons with other atoms. Single, double, and triple bonds are common, with carbon often central to these structures.
Reactivity and Periodicity
Carbon’s position in the periodic table informs its chemical properties. Its small atomic size and high electronegativity make it nonmetallic. It has an intermediate electronegativity compared to other elements, meaning it can both lose and gain electrons.
Molecular Bonding and Properties
Carbon’s tetrahedral shape in covalent bonding leads to the formation of hydrocarbons, organic compounds composed of only carbon and hydrogen. Different hydrocarbons have distinct properties, from the simple methane to the complex polyethylene.
Organic Life
Organic chemistry is the branch of chemistry that deals with carbon-based life. Carbon forms the backbone of amino acids, lipids, carbohydrates, and nucleic acids – the fundamental building blocks of living organisms. Its ability to form endless molecular structures allows for the diversity and complexity of life.
Valence Electrons and Chemical Bonding
- Describe the role of valence electrons in chemical bonding.
- Explain different types of chemical bonds involving carbon.
Valence Electrons and Chemical Bonding: The Dance of Carbon
Carbon, the magical element that underlies the very foundation of life, holds an arsenal of chemical tricks up its sleeve, orchestrated by its valence electrons. These electrons, the outermost electrons in carbon’s atomic shell, are the key players in the chemical bonds that define how carbon behaves and interacts with other elements.
The Crucial Role of Valence Electrons
- Valence electrons determine the chemical reactivity of an element.
- They decide how readily an element can gain, lose, or share electrons to form chemical bonds.
Types of Chemical Bonds Involving Carbon
- Covalent Bonds: Carbon shares its valence electrons with other atoms, forming pairs of shared electrons. These bonds are strong and stable, and they create the backbone of many organic molecules.
- Ionic Bonds: Carbon donates or accepts electrons to form ions (charged atoms). Ionic bonds are formed between metals and nonmetals, and they are typically weaker than covalent bonds.
- Metallic Bonds: Carbon atoms in a metal share all of their valence electrons, creating a “sea” of electrons that allows for the free flow of electricity.
Carbon’s Bonding Versatility
Carbon’s tetravalence (four valence electrons) makes it incredibly versatile in forming bonds. It can form single bonds, double bonds, or triple bonds, allowing it to create a wide range of molecules with diverse properties.
- Single Bonds: When carbon shares one pair of valence electrons with another atom.
- Double Bonds: When carbon shares two pairs of valence electrons with another atom.
- Triple Bonds: When carbon shares three pairs of valence electrons with another atom.
Valence electrons are the chemical dance partners of carbon, allowing it to form a vast array of bonds and create molecules with remarkable properties. These bonds are essential for the formation of organic molecules, the building blocks of life, and for the existence of carbon-based materials like graphite and diamond.
Periodic Properties and Reactivity: Carbon’s Place in the Chemical Canvas
In the grand tapestry of elements, carbon holds a captivating place. Its unique atomic structure, with a mere six electrons arranged around its tiny nucleus, grants it an extraordinary ability to form diverse chemical bonds. This versatility is the very essence of carbon’s remarkable properties and reactivity.
Carbon resides in Group 14 of the periodic table, a family of elements known as the carbon group. Its atomic number of 6 indicates the presence of six protons in its nucleus, while the electron configuration of 1s²2s²2p² reveals the arrangement of its electrons. These six electrons determine its position and characteristics within the periodic table.
Periodic trends, the gradual changes in properties across the periodic table, play a pivotal role in shaping carbon’s behavior. Atomic size increases down the group of elements, due to the addition of energy levels, and carbon lies at the sweet spot with a relatively small atomic size. This compact structure allows carbon to form strong and stable bonds with other elements.
Ionization energy, the energy required to remove an electron from an atom, increases across a period. Carbon’s position in the second period endows it with a higher ionization energy compared to elements in subsequent periods, indicating that it holds onto its electrons more tightly. This stability contributes to carbon’s ability to form covalent bonds, sharing electrons with other atoms to create molecules.
Electronegativity, the tendency of an atom to attract electrons, also follows periodic trends, increasing across a period. Carbon’s position in the second period results in a moderate electronegativity, enabling it to form polar covalent bonds, where the electrons are not evenly shared between the atoms. This polarity plays a crucial role in the formation of many organic compounds that are essential for life.
Thus, carbon’s unique electron configuration and atomic number influence its periodic position and properties, paving the way for the remarkable reactivity that has shaped the world as we know it.
Molecular Bonding and Chemical Properties of Carbon
Introduction:
Carbon, the foundation of all life on Earth, exhibits an unparalleled versatility in its chemical behavior. This extraordinary range stems from the unique bonding patterns it can form, offering a diverse array of molecular structures and properties.
Types of Molecular Bonds
Carbon’s valence electrons (four) enable it to form covalent bonds with itself or other atoms. Covalent bonds arise when atoms share electron pairs, forming a strong bond between them. Carbon can form single, double, or triple covalent bonds, creating a vast repertoire of molecular structures.
Influence of Molecular Structure on Chemical Properties
The arrangement of atoms within a molecule significantly influences its chemical properties. Polarity arises when electrons are unequally distributed within a molecule, resulting in oppositely charged regions. Polarity affects solubility, reactivity, and intermolecular interactions. Molecular shape also plays a crucial role, governing factors such as dipole moment, reactivity, and biological activity.
Polar Covalent Bonds and Electronegativity
When carbon bonds with atoms of different electronegativities, the resulting bond becomes polar. Electronegativity measures an atom’s ability to attract electrons towards itself. The difference in electronegativity determines the degree of polarity.
Hybridization and Bonding
Carbon can undergo hybridization, where atomic orbitals merge to form new hybrid orbitals with specific shapes and orientations. This process allows carbon to form various molecular geometries, such as tetrahedral, trigonal planar, and linear, each with its distinct bonding properties.
Conclusion:
The remarkable molecular bonding and chemical properties of carbon account for its ubiquitous presence in nature and its central role in life processes. From the intricate structures of biological molecules to the diverse applications of carbon materials, this element continues to inspire and fascinate scientists and researchers alike.
Organic Chemistry: The Chemistry of Carbon-Based Life
Carbon, the essential building block of life, plays a pivotal role in the intricate tapestry of biological systems. Organic chemistry is the fascinating branch of science that delves into the world of carbon-based compounds, unlocking the secrets of life’s molecular machinery.
Hydrocarbons: The Foundation of Organic Molecules
Organic molecules, the cornerstones of living organisms, are built upon a foundation of hydrocarbons. These compounds, consisting solely of carbon and hydrogen atoms, exhibit a myriad of structures and properties. From the simplest methane to the complex chains of polymers, hydrocarbons form the backbone of organic chemistry.
Functional Groups: The Chemical Alphabet
The chemical diversity of organic molecules arises from the presence of functional groups. These specific arrangements of atoms, such as -OH (hydroxyl) or -COOH (carboxyl), impart characteristic chemical properties to each compound. Functional groups act as the “letters of the chemical alphabet”, enabling organic molecules to interact and communicate with each other.
Importance in Biological Systems
Organic chemistry is essential for understanding the molecular basis of life. Carbohydrates, proteins, lipids, and nucleic acids, the fundamental building blocks of cells, are all organic compounds. Their intricate structures and interactions govern the cellular machinery that sustains life.
Organic chemistry unravels the secrets of life’s molecular tapestry. By understanding the chemistry of carbon-based molecules, we gain insights into the fundamental processes of biology and pave the way for advancements in medicine, biotechnology, and other fields that shape our world.
Special Forms of Carbon: Graphite and Its Versatile Applications
Carbon, the building block of life, exhibits remarkable properties that extend beyond its role in biological systems. One of its most fascinating forms is graphite, a layered mineral with extraordinary characteristics and wide-ranging applications.
Graphite’s unique structure consists of planes of carbon atoms arranged in a hexagonal lattice. These layers stack upon each other, held together by weak bonds. This arrangement grants graphite its lubricating properties and makes it an ideal material for use in pencils, dry lubricants, and other friction-reducing applications.
Beyond its role as a lubricant, graphite possesses several other valuable properties. It is an excellent conductor of electricity, making it suitable for use in batteries, electrodes, and electrical components. Its thermal conductivity also makes it useful for heat dissipation in electronic devices and heat exchange applications.
The versatility of graphite extends to its ability to form various derivatives, each with its own unique set of properties:
-
Carbon nanotubes: Hollow, cylindrical structures formed by rolling up a graphene sheet.
- They possess exceptional electrical and thermal conductivity and are used in electronics, nanotechnology, and composites.
- Example: They are used in high-performance transistors and batteries.
-
Carbon fibers: Strong, lightweight fibers spun from graphite filaments.
- They offer high tensile strength and rigidity and are used in aerospace, automotive, and sporting equipment applications.
- Example: They are found in rocket bodies, aircraft fuselages, and racing car chassis.
-
Graphene: A single layer of graphite, the thinnest and strongest known material.
- It exhibits remarkable electrical, thermal, and mechanical properties and has potential applications in electronics, energy storage, and composites.
- Example: It could revolutionize solar cells and energy-efficient lighting.
-
Carbon black: A fine powder composed of tiny, spherical carbon particles.
- It is used as a pigment in inks, paints, and plastics and also finds applications in batteries, rubber products, and conductive coatings.
- Example: It is responsible for the black color of printer ink and tire sidewalls.
The diverse properties and applications of graphite and its derivatives make it an essential material in various industries, including engineering, electronics, energy, and manufacturing. Its unique structure and versatility continue to inspire innovation and hold promise for future advancements.
Diamond: The Invincible Gemstone
In the realm of natural wonders, diamond stands apart as the hardest known substance, a testament to its unparalleled strength and resilience. Its crystalline structure, an intricate lattice of tightly bound carbon atoms, is responsible for this exceptional durability.
This carbon-based wonder is not only a symbol of beauty and wealth but also a highly valuable industrial material. Its resistance to wear and tear makes it the ideal choice for cutting tools, particularly in the precision manufacturing of delicate components. Diamond’s exceptional thermal conductivity, even surpassing that of metals, allows for efficient heat dissipation, making it suitable for applications where cooling is crucial.
The allure of diamond extends beyond its practical uses. Its iridescence and fire have captivated humans for centuries, making it a coveted gemstone. From the legendary Hope Diamond to the Koh-i-Noor, diamonds adorn crowns, necklaces, and earrings, symbolizing power, status, and enduring love.
Unlocking the Mysteries of Diamond
The formation of diamonds is a captivating story of extreme temperature and pressure. Deep within the Earth’s mantle, under conditions of immense heat and compression, carbon atoms arrange themselves in a rigid crystalline structure, creating the diamond. This process can take millions of years, giving rise to the oldest and most enduring material on our planet.
Diamond’s Diverse Applications
Beyond its use in jewelry and cutting tools, diamond’s unique properties have led to its application in a wide range of fields. In electronics, diamond is used as a semiconductor and heat sink. It also plays a crucial role in the development of quantum computing, where its defect-free structure holds the promise of revolutionary computational power.
The medical industry has also embraced diamond’s exceptional qualities. Its biocompatibility and resistance to wear make it ideal for surgical instruments and implants. Diamond-coated stents and hip replacements can extend the lifespan of these devices and improve patient outcomes.
Diamond, nature’s marvel, embodies strength, beauty, and practicality. Its unique crystalline structure grants it a multitude of applications, from exquisite jewelry to cutting-edge technology. As we continue to unravel the secrets of this remarkable gemstone, its role in shaping our world will undoubtedly grow.
Carbon Dating: Unlocking the Past
Carbon, the versatile element that forms the backbone of life, also holds the secrets to our ancient history. Carbon dating, a remarkable scientific technique, utilizes the power of this element to unveil the age of archaeological artifacts, geological formations, and paleontological remains.
The principle behind carbon dating lies in the presence of two isotopes of carbon: carbon-12 and carbon-14. While carbon-12 is stable, carbon-14 is radioactive, decaying at a constant rate over time. Living organisms contain both isotopes in a specific ratio, but upon death, the uptake of carbon-14 ceases. This sets in motion a radioactive decay process that gradually reduces the amount of carbon-14 relative to carbon-12.
By analyzing the ratio of carbon-14 to carbon-12 in a sample, scientists can determine how much time has passed since the organism died or the artifact was created. This technique has revolutionized our understanding of the past, allowing us to date archaeological remains, fossils, and even ancient climates with remarkable accuracy.
In archaeology, carbon dating has illuminated the chronology of human civilization, providing valuable insights into our ancestors’ cultural and technological advancements. From the pyramids of Egypt to the cave paintings of Lascaux, carbon dating has helped us piece together the story of our human heritage.
In geology, carbon dating has aided in understanding the formation of ancient rock formations, unraveling the Earth’s geological history. From the layering of sedimentary rocks to the dating of volcanic eruptions, carbon dating has provided a timeline for the Earth’s dynamic past.
In paleontology, carbon dating has illuminated the timeline of life on Earth, helping scientists understand the evolution and extinction of species. From the dating of dinosaur fossils to the reconstruction of prehistoric ecosystems, carbon dating has played a pivotal role in our understanding of Earth’s biological history.
Carbon dating, a testament to the power of science, continues to unlock the secrets of our past, providing invaluable insights into the history of life, the Earth, and human civilization.