Understanding Atom Properties And Their Impact On Chemical Behavior
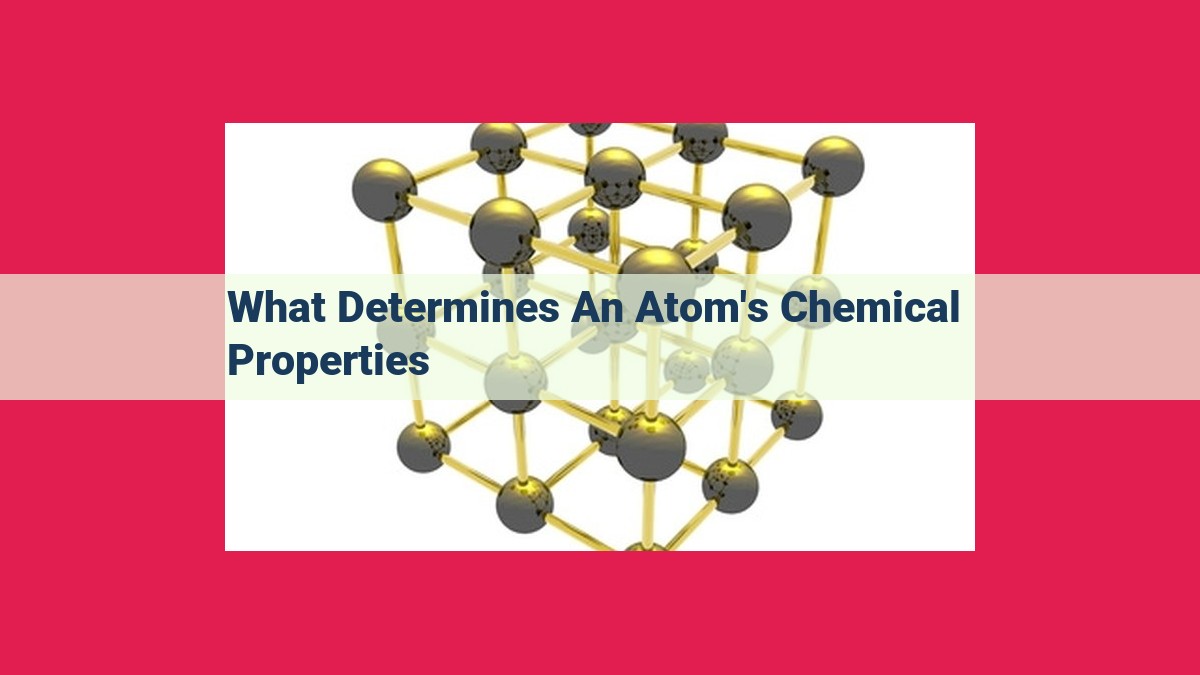
An atom’s chemical properties stem from its electron configuration and nuclear charge. The number of protons, known as the atomic number, determines the element’s identity and its electronic structure. Valence electrons, residing in the outermost shell, play a crucial role in chemical bonding by interacting with other atoms. The nuclear charge attracts electrons, influencing atomic size and reactivity. Ionization energy and electron affinity measure the ease of electron loss and gain, respectively, and correlate with atomic and molecular interactions. Electronegativity reflects the atom’s tendency to attract electrons, affecting bond polarity and molecular shape. Finally, atomic radius, the distance from the nucleus to the outermost electron shell, governs atomic and molecular interactions.
The Significance of Atomic Number: The Cornerstone of Element Identity
In the fascinating realm of chemistry, atomic number holds sway as a pivotal concept that defines the very essence of an element. It’s the unique numeric identifier that sets apart each element from its peers on the periodic table, establishing its chemical identity and dictating its distinctive properties.
The Atomic Number: Unveiling the Essence of Elements
The atomic number of an element represents the number of protons residing within the nucleus of its atoms. Protons, along with neutrons, form the dense core of an atom, while electrons whirl about in their designated energy levels. It is the number of protons that truly sets one element apart from another.
Atomic Mass: A Reflection of Both Protons and Neutrons
Closely entwined with the atomic number is the concept of atomic mass. While the atomic number indicates the proton count, the atomic mass encompasses both protons and neutrons. Neutrons are subatomic particles found within the nucleus alongside protons, contributing to the overall mass of an atom.
Isotopes: Variations Within the Same Element
Fascinatingly, elements can exhibit different atomic masses despite sharing the same atomic number. These variations arise from the existence of isotopes. Isotopes are atoms of the same element that share the identical number of protons but differ in their neutron count. As a result, isotopes of the same element exhibit distinct atomic masses.
For instance, carbon, an element with an atomic number of 6, has three common isotopes: carbon-12, carbon-13, and carbon-14. Each isotope contains 6 protons, but they differ in their neutron counts, leading to varying atomic masses.
Electron Configuration: The Blueprint of Atomic Identity
Within the intricate realm of atomic structure, one concept stands out as a fundamental building block: electron configuration. It unveils the distribution of electrons, the negatively charged particles that dance around the positively charged nucleus of an atom. This arrangement plays a pivotal role in dictating an element’s chemical reactivity, the driving force behind its interactions with other atoms.
Delving into Electron Orbitals
Electrons occupy specific regions around the nucleus, known as orbitals. Each orbital is characterized by its unique shape, energy level, and spin. Together, these properties form a hierarchy of orbitals, with the lowest energy levels being filled first according to the aufbau principle. The outermost orbitals, called valence orbitals, contain the electrons involved in chemical reactions, making them the gatekeepers of an atom’s reactivity.
Quantum Numbers: Describing the Electron’s World
To fully understand electron configuration, we must introduce a set of quantum numbers. These numbers describe the properties of each electron within an orbital:
- Principal quantum number (n): Determines the energy level of the orbital.
- Azimuthal quantum number (l): Defines the shape of the orbital.
- Magnetic quantum number (ml): Specifies the orientation of the orbital in space.
- Spin quantum number (ms): Indicates the spin direction of the electron.
These quantum numbers work in concert to create a “fingerprint” for each electron, providing a detailed map of its atomic abode.
Valence Electrons: The Bonding Masterminds
In the bustling metropolis of the atomic world, valence electrons are the vibrant and sociable inhabitants that determine the chemistry of elements. These electrons reside in the outermost shell of an atom, like extroverts eager to interact with the world around them.
Valence electrons play a pivotal role in bonding behavior, the way atoms join hands to form molecules and compounds. They are the social butterflies of the atomic world, determining an element’s chemical reactivity. Elements with a high number of valence electrons are more willing to share or gain electrons, making them highly reactive. On the other hand, elements with a low number of valence electrons are more reluctant to interact, resulting in lower reactivity.
Imagine a lively party where valence electrons are the guests. Some electrons are outgoing and eager to dance with other atoms’ electrons, forming covalent bonds. These bonds are like strong friendships, with electrons happily sharing their space to create stable molecules. Other electrons prefer to be more reserved, accepting electrons from neighboring atoms to form ionic bonds. These bonds resemble one-sided relationships, with the electron-accepting atom gaining a negative charge and the electron-donating atom becoming positively charged.
The number of valence electrons also influences the electronegativity of an element, which measures its ability to attract electrons towards itself. The more valence electrons an element has, the less electronegative it is. This can be compared to the popularity of a social gathering; elements with many valence electrons are like popular hosts who draw a crowd of electrons, while elements with fewer valence electrons are less attractive.
In summary, valence electrons are the driving force behind chemical bonding. Their number and behavior determine an element’s reactivity, bonding tendencies, and other atomic properties. They are the architects of the microscopic world, shaping the chemical landscapes that govern our existence.
The Attraction Factor: Nuclear Charge and Its Influence
Amidst the vastness of the atomic realm, where infinitesimal particles dance in unison, there lies a fundamental force that governs their behavior: nuclear charge. This enigmatic property, embedded within the core of every atom, exerts an invisible but powerful influence, shaping the very essence of atomic matter.
The nuclear charge, also known as the atomic number, represents the number of protons residing within the atom’s nucleus. These positively charged particles, along with the neutral neutrons, constitute the atom’s dense, central region. The nuclear charge plays a pivotal role in determining the atom’s identity, dictating which element it belongs to on the periodic table.
Moreover, the nuclear charge exerts a profound influence on the atom’s size and radioactivity. The greater the nuclear charge, the more protons are packed within the nucleus, creating a stronger electrostatic attraction for the surrounding electrons. This attraction draws the electrons inward, reducing the atom’s overall size. Conversely, atoms with fewer protons possess weaker nuclear charges, resulting in larger atomic radii.
Furthermore, the nuclear charge has a direct bearing on an atom’s propensity for radioactivity. Unstable isotopes, characterized by an excess of neutrons relative to protons, undergo radioactive decay to achieve a more stable nuclear configuration. The stronger the nuclear charge, the more stable the nucleus becomes, making it less likely to undergo decay.
In essence, the nuclear charge serves as a cornerstone of atomic structure and behavior, influencing everything from the element’s identity to its physical properties and radioactive stability. Understanding this fundamental property provides a deeper insight into the intricate workings of the atomic realm.
Ionization Energy: A Measure of Electron Loss
In the enigmatic world of atomic structure, the ionization energy stands as a crucial measure of an element’s disposition to relinquish its electrons. This intriguing property reveals the tenacity with which atoms cling to their negatively charged particles, holding them captive within their electronic embrace. But what is ionization energy, and why is it so significant? Join us as we embark on a captivating journey to unravel the secrets behind this fascinating atomic attribute.
Ionization energy, expressed in units of electronvolts (eV), represents the minimum amount of energy required to dislodge an electron from the outermost shell of a gaseous atom. This process, known as ionization, effectively transforms the atom into a positively charged ion. The greater the ionization energy, the more strongly the atom holds onto its electrons, exhibiting a higher resistance to ionization.
The ionization energy of an element is directly correlated with its atomic radius. Simply put, the larger the atom, the more diffuse its electron cloud, making it easier to remove an electron. This relationship stems from the reduced electrostatic attraction between the positively charged nucleus and the outermost electrons in larger atoms. Conversely, smaller atoms possess a more compact electron cloud, resulting in a stronger attractive force and a higher ionization energy.
Another captivating connection exists between ionization energy and electronegativity. Electronegativity measures an atom’s ability to attract electrons towards itself. Elements with high electronegativity, such as fluorine and oxygen, exhibit a strong pull on electrons, making it difficult to remove them and resulting in high ionization energies. In contrast, elements with low electronegativity, like sodium and potassium, have a weaker attraction for electrons, leading to lower ionization energies.
Comprehension of ionization energy is essential for unraveling the intricate tapestry of chemical reactions and bonding. It influences the formation of ions, the stability of compounds, and the reactivity of elements. By delving into the depths of ionization energy, we gain invaluable insights into the fundamental principles governing the behavior of matter on an atomic level.
Electron Affinity: A Gain to Consider
In the realm of atomic chemistry, electron affinity stands as a crucial factor that determines the inclination of atoms to attract and hold additional electrons. This property, measured in kilojoules per mole (kJ/mol), reveals the energy change that occurs when an atom gains an electron.
Unlike ionization energy, which measures the energy required to remove an electron, electron affinity quantifies the energy released when an electron is added. This energy can be positive or negative, signifying whether the process is exothermic (energy is released) or endothermic (energy is absorbed), respectively.
Atoms tend to exhibit higher electron affinity when they have a high effective nuclear charge and a low number of electrons. This is because more positively charged nuclei attract electrons more strongly. Conversely, atoms with a large number of electrons experience increased electron-electron repulsion, making it harder to add additional electrons.
The concept of electron affinity is pivotal in understanding chemical bonding. When two atoms with different electron affinities interact, the atom with the higher electron affinity will attract the shared electrons more strongly. This creates a shift in electron density, polarizing the bond. The electronegativity of atoms, which is closely related to electron affinity, plays a significant role in determining bond polarity.
Electron affinity also influences redox reactions. In reduction reactions, atoms tend to gain electrons, resulting in a decrease in energy. The greater the electron affinity, the more favorable the reduction process. Conversely, in oxidation reactions, atoms lose electrons, and electron affinity exerts an influence on the difficulty of the process.
Electronegativity: The Balancing Act
Electronegativity, a fundamental chemical property, plays a pivotal role in determining the behavior of atoms and molecules. Defined as the ability of an atom to attract electrons towards itself, electronegativity governs the polarity of chemical bonds and ultimately shapes the geometry of molecules.
Imagine a chemical bond as a tug-of-war between atoms. When atoms have significantly different electronegativities, the more electronegative atom exerts a stronger pull on the shared electrons, creating an unequal distribution. This asymmetry in electron distribution results in polar covalent bonds, where one end of the bond carries a partial positive charge and the other a partial negative charge.
The electronegativity of an atom influences not only bond polarity but also molecular geometry. Highly electronegative atoms tend to pull electrons towards themselves, resulting in a more compact and symmetrical molecular structure. For instance, in water (Hâ‚‚O), the highly electronegative oxygen atom draws the electrons towards itself, creating a bent molecular shape known as a tetrahedron.
Understanding electronegativity is crucial for predicting the properties of various compounds. For example, it helps explain why some materials are good electrical conductors while others are insulators. Metals, with their low electronegativity, readily give up electrons, enabling them to flow freely and conduct electricity. In contrast, nonmetals, with their high electronegativity, hold onto their electrons tightly, making them poor conductors.
In summary, electronegativity is a fundamental property that governs the polarity of chemical bonds and the geometry of molecules. It plays a crucial role in determining the behavior and properties of a wide range of chemical substances, from simple molecules to complex materials.
Atomic Radius: The Measure of Space
- Explanation of atomic radius and its effects on atomic and molecular interactions
- Distinctions between ionic, covalent, and metallic radii
Atomic Radius: The Measure of Space
Imagine yourself as a chemist, embarking on a journey into the fascinating realm of atoms. In this microscopic world, the atomic radius plays a pivotal role, shaping the behavior and interactions of these tiny particles. Let’s delve into its intriguing nature and explore its profound implications.
The atomic radius is the measure of the distance from the nucleus (the heart of the atom) to the outermost electron shell. This crucial parameter determines the size of an atom and influences its interactions with other atoms. It also affects properties such as atomic volume, density, and surface area.
There are three main types of atomic radii:
- Ionic radius: This is the radius of an atom when it has gained or lost electrons, forming an ion.
- Covalent radius: This is the radius of an atom when it is covalently bonded to another atom.
- Metallic radius: This is the radius of an atom in a metal, which is characterized by the free movement of electrons.
The type of atomic radius depends on factors such as the number of protons and electrons in the atom, the number of shells, and the type of bonding. Elements with more electrons and fewer protons tend to have larger atomic radii.
Understanding the atomic radius is essential for comprehending chemical reactions and properties. For instance, atoms with larger atomic radii are more reactive than those with smaller radii. This is because atoms with larger atomic radii have their outermost electrons farther from the nucleus, making them easier to remove or share.
Moreover, the atomic radius influences the packing of atoms in solids, the formation of molecules, and the properties of materials. In summary, the atomic radius is a fundamental concept that provides insight into the structure and behavior of matter at its very core.