Amino Acid Bonding In Protein Synthesis: A Comprehensive Guide
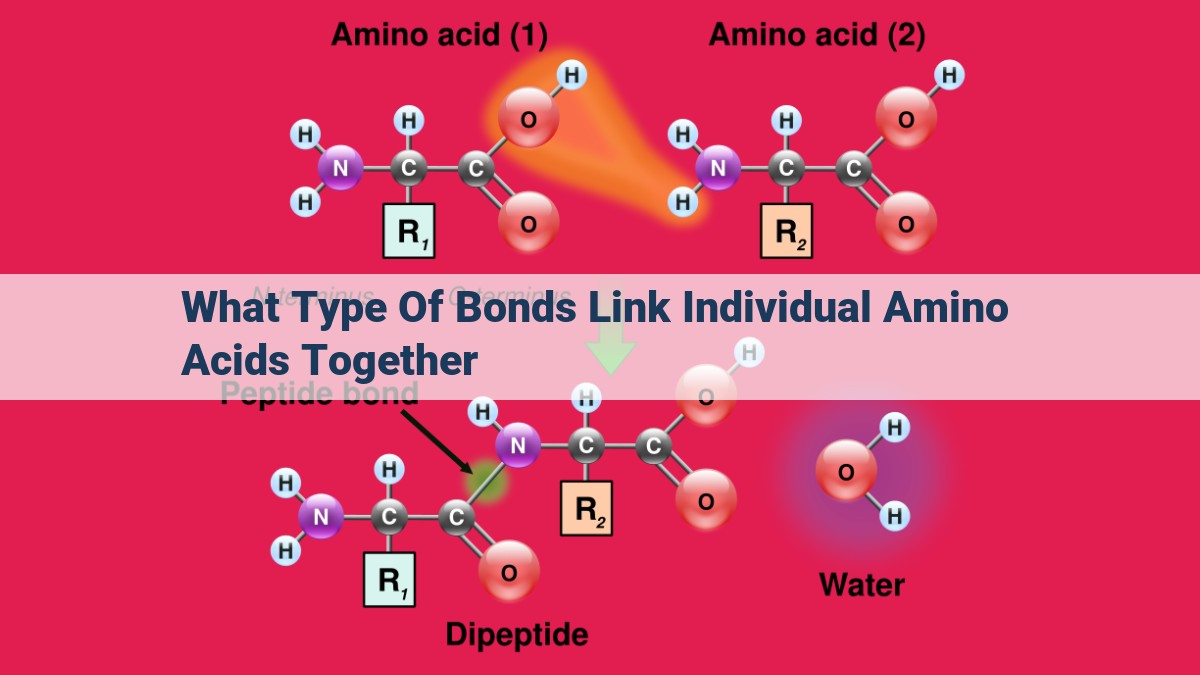
Individual amino acids are linked together by peptide bonds formed through dehydration synthesis during protein synthesis. Peptide bonds connect the amino group of one amino acid to the carboxylic acid group of another, forming a polypeptide chain. These chains are further stabilized by hydrogen bonds, van der Waals forces, and disulfide bonds. Glycosidic bonds also play a role in some proteins, linking carbohydrates to the amino acid side chains.
Peptide Bonds: The Foundation of Protein Chains
In the bustling metropolis of the cell, there exists a remarkable building block—the peptide bond. This tiny molecular link plays a crucial role in constructing the intricate structures of proteins, the workhorses of our bodies.
Picture a series of amino acids, essential building blocks of proteins, lining up like a row of beads. As the cell prepares to assemble a new protein, a chemical dance unfolds. Each amino acid’s amine group reaches out, grasping the carboxylic acid group of its neighbor. As they embrace, a water molecule is expelled, creating a covalent bond—the peptide bond.
This dehydration synthesis process continues, bead by bead, until a long polypeptide chain emerges. These polypeptide chains are the blueprints for proteins, dictating their shape and function. They can twist into helices, fold into sheets, or form intricate networks, creating the diverse array of proteins that enable life.
Peptide bonds are not merely inert connections; they are fundamental to the structure, function, and diversity of proteins. They provide the foundation upon which our bodies build, repair, and thrive.
Hydrogen Bonds: Sculpting the Protein’s Shape
- Description of hydrogen bond formation and their role in protein structures
- Explanation of alpha helix and beta sheet conformations
Hydrogen Bonds: The Sculptors of Protein Shape
In the intricate tapestry of life’s molecules, proteins stand as masterful architects, shaping the very fabric of our cells. At the core of these molecular marvels lies a fundamental force that orchestrates their intricate structures: the humble hydrogen bond.
The Invisible Glue: Hydrogen Bonds in Action
Hydrogen bonds arise from the electrostatic attraction between a hydrogen atom and an electronegative atom, such as oxygen or nitrogen. In proteins, these bonds form between polar amino acids, such as glycine and alanine, and water molecules or other polar groups.
Think of hydrogen bonds as tiny magnets, subtly pulling atoms together. They’re not covalent bonds, but they’re strong enough to influence the overall shape and stability of proteins.
Alpha Helix: The Springy Spiral
One of the most prevalent protein structures is the alpha helix. Imagine a spiral staircase, with its amino acids winding around a central axis. Hydrogen bonds stabilize this helical shape, forming between the backbone nitrogen and carbonyl oxygen of consecutive amino acids.
Beta Sheet: The Parallel Planes
In contrast to the alpha helix, the beta sheet is a planar structure. Hydrogen bonds form between the backbone nitrogen and carbonyl oxygen of different strands, creating parallel sheets. These structures are often involved in protein-protein interactions.
The Dance of Conformity: Shaping Protein Function
Hydrogen bonds are not merely passive spectators; they actively shape the function of proteins. By influencing the protein’s shape, they control its ability to bind to ligands, catalyze reactions, and interact with other molecules.
For instance, the shape of an enzyme’s active site is determined by hydrogen bonds between amino acids. This precise shape ensures that the enzyme can only bind to specific substrates, facilitating specific chemical reactions.
Hydrogen bonds are the unsung heroes of protein architecture. These invisible forces shape the intricate structures of proteins, dictating their function and ultimately influencing the very processes of life. Understanding the role of hydrogen bonds is crucial for unraveling the mysteries of protein function and paving the way for new discoveries in biochemistry and medicine.
Van der Waals Forces: The Glue that Keeps Proteins in Shape
Imagine tiny, invisible magnets scattered throughout a protein molecule. These magnetic forces, known as van der Waals forces, play a crucial role in stabilizing the protein’s intricate 3D shape. They are the subtle interactions that keep proteins from falling apart.
Van der Waals forces are weak attractions that arise from the uneven distribution of electrons within molecules. These forces are present in all molecules, but they become especially significant in large molecules like proteins, where numerous atoms can interact.
Their Relevance in Tertiary Structure Formation:
As proteins fold into their functional shapes, van der Waals forces help guide the formation of the tertiary structure. This level of protein structure refers to the distinctive shape of a single polypeptide chain. Whether it forms a compact ball, an elongated helix, or a complex meshwork depends heavily on these subtle interactions.
Van der Waals forces favor the alignment of nonpolar (hydrophobic) amino acid side chains towards the interior of the protein, creating a hydrophobic core. This arrangement shields the hydrophobic side chains from the surrounding aqueous environment, contributing to the protein’s overall stability.
In contrast, polar (hydrophilic) amino acid side chains tend to be found on the surface of the protein, where they interact with water molecules. This segregation of hydrophilic and hydrophobic regions is a key factor in the folding and stability of proteins.
Without the stabilizing force of van der Waals interactions, proteins would be unable to maintain their precise shapes, which are essential for proper function. These subtle forces act as the glue that holds proteins together, enabling them to perform the vital tasks that keep our bodies running smoothly.
Disulfide Bonds: The Covalent Bridges Enhancing Protein Structure
In the realm of proteins, structure plays a pivotal role in their function. One of the key players in shaping and stabilizing protein architecture is the disulfide bond, a covalent bridge formed between cysteine molecules.
Disulfide bonds arise when two cysteine residues, each containing a thiol (-SH) group, undergo an oxidation-reduction reaction. During this process, one cysteine loses a hydrogen atom, becoming a cysteine thiolate anion, while the other gains a hydrogen atom, becoming a cysteine sulfenic acid. These two intermediates then react to form a disulfide bond, connecting the two cysteines via a covalent sulfur-sulfur bond.
Disulfide bonds have a remarkable ability to stabilize protein structure by preventing polypeptide chains from unfolding or unraveling. They act as rigid cross-links, locking different regions of the protein in place. This structural integrity is crucial for proteins to carry out their biological functions effectively.
In the tertiary structure of a protein, disulfide bonds play a particularly important role. They connect distant parts of the polypeptide chain, bringing them closer together and forming loops or domains. This intricate network of disulfide bonds contributes to the overall compactness and stability of the protein’s three-dimensional shape.
In some proteins, disulfide bonds also contribute to the quaternary structure, which refers to the assembly of multiple polypeptide chains into a functional complex. By linking different subunits together, disulfide bonds ensure the proper arrangement and coordination of these subunits, allowing the protein complex to function as a cohesive unit.
In conclusion, disulfide bonds are essential covalent bridges that enhance the structural stability and integrity of proteins. They play a vital role in shaping the three-dimensional architecture of proteins, ensuring their proper function and maintaining their biological activity.
Glycosidic Bonds: Linking Carbohydrates to Proteins
- Definition of glycosidic bonds and their formation
- Discussion of the role of glycosidic bonds in glycoprotein creation and cellular functions
Glycosidic Bonds: The Sweet Connection Between Carbohydrates and Proteins
In the intricate dance of life’s molecules, glycosidic bonds play a vital role in bridging the gap between carbohydrates and proteins, creating a harmonious symphony of structure and function. These chemical bonds, formed through the union of a carbohydrate molecule with an alcohol group on an amino acid, are the foundation of glycoproteins, a class of molecules that perform a myriad of crucial roles in our bodies.
Forming the Glycosidic Union
Imagine a carbohydrate molecule, its saccharine sweetness ready to embrace another component. It encounters a hydroxyl group on the side chain of an amino acid, such as serine or threonine. Like two puzzle pieces fitting together, the carbohydrate and hydroxyl group undergo a dehydration reaction, releasing a molecule of water. In this dance of atoms, a glycosidic bond is forged, linking the carbohydrate to the amino acid.
Glycoproteins: More Than Meets the Eye
Glycoproteins are not mere spectators in the cellular ballet; they are active participants in a wide range of biological processes. They adorn cell surfaces, acting as identity markers that guide interactions between cells. They facilitate nutrient absorption, escorting molecules across cell membranes. And they play a critical role in the immune system, helping to recognize and neutralize foreign invaders.
Unveiling the Cellular Symphony
The role of glycosidic bonds in glycoprotein function extends beyond their structural backbone. These bonds determine the carbohydrate’s molecular shape and composition, which in turn influences the glycoprotein’s interactions with other molecules. This elegant interplay of structure and function is essential for coordinating the intricate symphony of life.
Glycosidic bonds, the intricate bridges between carbohydrates and proteins, are the unsung heroes of our cells. They orchestrate the creation of glycoproteins, molecules that are essential for our survival and well-being. By understanding the delicate dance of these bonds, we gain a deeper appreciation for the remarkable complexity and harmony of life’s molecular machinery.